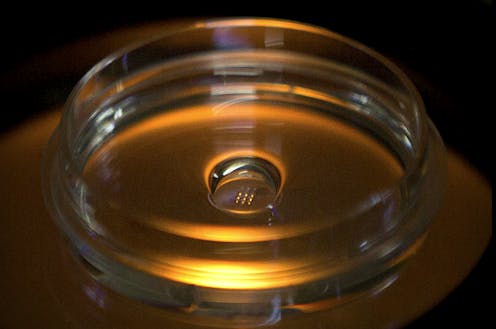
Consider a living cell, which can have thousands of genes. Now think of these genes as dials that can be tweaked to change how the cell grows in a given environment. Tweaking a gene can either increase or decrease growth, and this is made more complex considering these dials are interconnected with each other, like cogs in a machine.
While scientists are now able to edit genes in laboratory conditions and attempt to produce findings that may lead to cures, evolution has been doing this for billions of years. Evolution is the natural process that turns these dials, allowing populations to adapt. However, unlike scientists, evolution turns these dials randomly as mutations affect the function of genes.
One underlying hypothesis in evolutionary theory — the evolutionary contingency hypothesis — has been that this tuning can have chaotic behaviours. Or, in other words, dials tweaked early in the process can dramatically alter later evolutionary potential.
Stephen Jay Gould was a famous proponent of this theory, arguing in his 1989 book Wonderful Life that since beneficial mutations occur randomly, chance must play an important role in evolutionary diversification.
Read more: Does our DNA really determine our intelligence and health?
If this hypothesis is true, it affects how scientists should edit genes in the laboratory as they will face the chaotic interconnections of our cells. Our work set out to test this hypothesis.
Resolving an evolutionary paradox
We can observe the process of evolution in the laboratory under extremely well-controlled conditions. We have done so by growing populations of micro-organisms for hundreds — even thousands — of days.
Since these organisms divide and reproduce so quickly, this process represents thousands of generations of growth. These experiments have allowed us to pinpoint precisely when, and how, beneficial mutations co-occur and compete to take over the population.
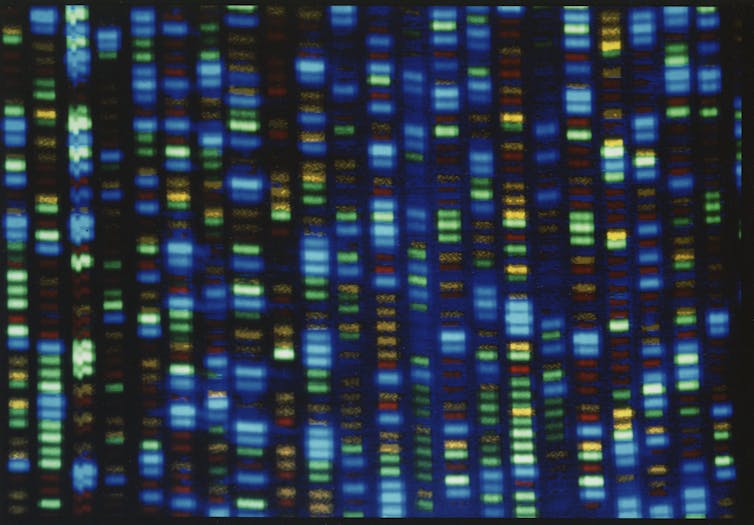
One striking observation from every single one of these experiments is that increases in fitness slow down over time at a rate that is surprisingly reproducible. Despite accumulating different mutations, different populations show remarkably predictable diminishing returns in how fast they adapt.
In contrast with the seemingly chaotic behaviour of mutations, fitness or growth changes are highly predictable. This has led many to hypothesize that this order of mutation is an inherent consequence of the way biological systems have evolved.
This striking hypothesis is at odds with the idea that the specifics of an organism’s biology matter for evolution. In other words, it has been difficult to prove that the order in which evolution turns dials has any impact on the future.
The answer to the paradox
My team was able to show that the answer to resolving this paradox lies within the interconnected gene network of the cell itself.
For evolution to work, the dial-tuning must be precise: even if the net outcome is beneficial, adjusting one set of linked dials can trickle down and affect other previously correctly placed dials. As evolution continues, the probability of breaking harmoniously-tuned dials grows. This seemingly simple principle explains why the rate of evolutionary improvements typically slows down over time.
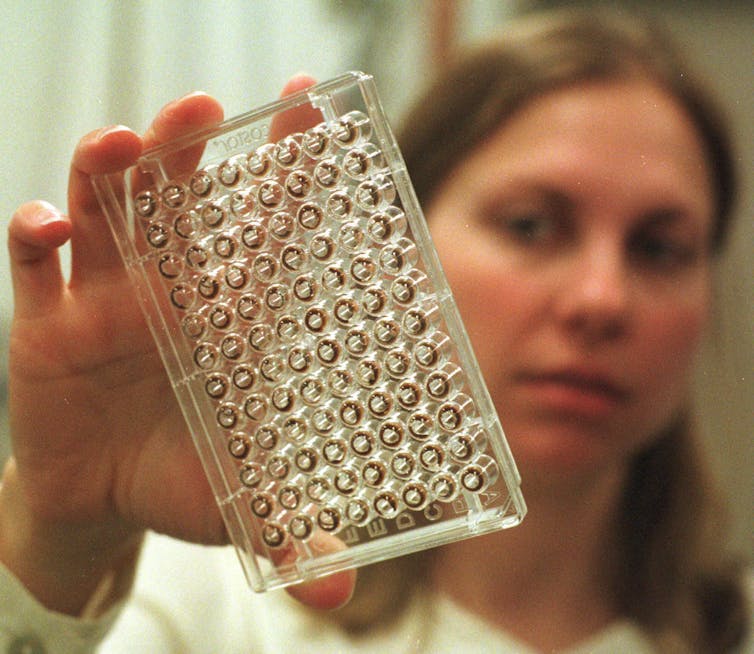
Resolving this paradox experimentally was not an easy task. After all, how can one show the entanglement of dials within the cell? In our recent study, we tackled this challenge by systematically trying out every possible combination of 10 key beneficial mutations and looking at how they affect the growth of cells.
By testing out combinations of mutations, we were able to reliably understand which mutations were entangled together (this entanglement is known as epistasis) and for just 10 mutations, over 1,000 combinations had to be generated.
How this affects genetic precision medicine
Current futuristic technologies tout the ability to generate precise single mutations within our own genomes with the hope that this can be used to repair non-functional genetic variants. For example, prime editing is an effective “search-and-replace” genome editing technology.
One important concern with these approaches is they can introduce undesired mutations at the same time. However, even as scientists solve these concerns, the field of human genetics has often overlooked the importance of the interconnectedness of genes.
Our study demonstrates that bioengineers should think not only about the effect a mutation has on the gene it is in, but also about the effect of the mutation in the context of all other variations in our genomes. Altering the function of any of our genes can affect our interconnected cellular networks.
This is compounded by the fact that all of us carry hundreds of extremely rare variants, which means each of us carries a unique interconnected network of genes. These personalized networks make us who we are.
Genome interpretation is at the heart of genetic testing for disease. And while scientists have made some progress in identifying key pathogenic genetic variants (those that can cause disease), our findings demonstrate that classifying a variant as pathogenic or benign requires us to also understand how the other genetic dials in our cells are tuned.

Alex Nguyen Ba receives funding from the Natural Sciences and Engineering Research Council of Canada.
This article was originally published on The Conversation. Read the original article.