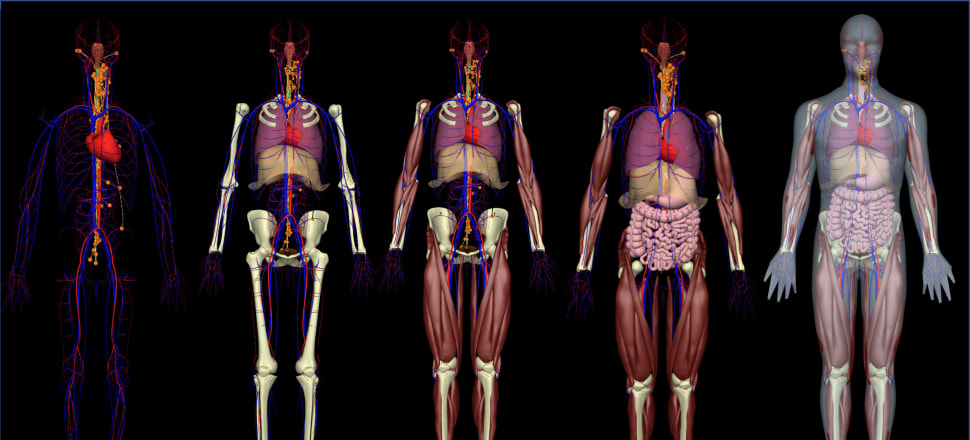
The concept of ‘digital twins’ for engineering systems has been around for years, and the same principles can be applied to human health
Opinion: When an aircraft takes off on an international flight, its jet engines are under maximum stress – the perfect time to be measuring as much as possible about how it is performing to predict any impending failure. In fact, that is exactly what happens, and those measurements are used to schedule any required maintenance when the plane lands at its destination.
The measurements are used with a mathematical model of the engine that includes all aspects of the mechanical, electrical and chemical processes needed to describe the function of the engine. The model is called a ‘digital twin’ of the engine because it mimics every aspect of the engine. Moreover, the parameters of the model are specific to that particular engine and kept up to date by the diagnostic measurements on the engine during take-off.
READ MORE:
* Now is the time to embed research into NZ’s healthcare system
* Finally, some good news about Parkinson’s disease
* Protect yourself with 'nocebo' knowledge
New engines are also designed and tested on a computer before construction starts because the laws of physics, embedded in the model, can accurately predict how the engine will behave. Similarly, for the whole aircraft, which can be ‘flown’ in the computer long before it is built. All complex engineering systems, including cars and cell phones, are designed and tested with computer models before they are built.
So what about a human digital twin? Could we not have a model of our own bodies, updated by regular diagnostic testing (under stressed conditions, such as exercise) and, given our particular genetic makeup and environment influences, used to prevent adverse consequences of inherited or acquired traits? Perhaps it could also be used – if needed – to help design optimal therapeutic interventions for an individual.
Biology is clearly extremely complex but it too has to obey the laws of physics and chemistry, so there is no fundamental reason why we could not build a predictive model of the anatomy and physiology of a human body capable of being personalised and used for disease prevention or treatment. That is exactly the goal of the Virtual Physiological Human or Physiome project, which when applied to an individual person in a medical context becomes the human ‘digital twin’.
There are two key differences between engineering systems (such as aircraft engines) and human physiology; one that makes the challenge easier and one that makes it a lot harder.
Diseases and drugs operate at the molecular scale, but with effects felt at the scale of tissues, organs and organ systems. At the bottom of this hierarchy is the genome – the code from which proteins and their regulatory systems are built. The good news is we know this code and can measure the small coding variations that give rise to the differences between people.
Thanks to the AI project AlphaFold we also know the structure of most proteins. This provides an extraordinary advantage over engineering systems, which are not able to benefit from such a clear understanding of the structure and properties of their component parts at the atomic scale.
A key aspect of a human digital twin is that … it is personalised as much as possible to an individual and continually updated with new data as new measurements are performed on that individual
The other key difference, and the one that makes modelling biological systems so much harder than modelling engineering systems, is that cells and tissues are continually growing and adapting to their environment. Our bodies are full of sensors generating signals that regulate the expression of new proteins and hence the tissue properties and therefore whole-body function. So, unlike most engineering systems, the material properties of the component parts of our bodies are dynamic.
Disease and degeneration (including ageing) happen at the molecular scale, but those changes are felt in the cells, tissues, organs, and whole-body organ systems that provide the physiological function of the body. When clinicians diagnose a chronic condition, they are often trying to make sense of data from all of these scales. Magnetic resonance imaging and computerised tomography scans, for example, provide insights into organ function, such as how the lungs are breathing or the heart is contracting.
Physiological tests, such as lung or heart function tests, provide data on gas exchange or cardiac output, often under exercise conditions. Blood tests are hugely important for monitoring biomarkers characteristic of tissue function or dysfunction. Genetic tests indicate familial predisposition to certain conditions, especially for rare diseases.
Because these multi-scale systems are so complex, mathematical models of the anatomy and physiology of the body, based on biophysical mechanisms and bridging spatial scales from genes and proteins to cells, tissues, organs and the whole body, can be enormously useful in making sense of the disparate clinical data – in exactly the same way multi-scale, physics-based models of engineering systems are essential to the understanding (and monitoring) of everything from aircraft and their engines down to cars and cell phones.
It is, however, important to acknowledge that while 50 years of research by molecular and cell biologists have given us a phenomenal picture of how cells and tissues work, the physiology of the body is hugely complex and there are many gaps in our knowledge. We now know a great deal about the DNA code for the approximately 20,000 mammalian genes and the structure of their proteins, but this represents only 2 percent of the genome. A good fraction of the other 98 percent encodes RNAs that regulate the expression of these proteins via transcriptional control mechanisms that we are only beginning to understand.
On the other hand, a great deal is known about physiological processes and how the body maintains the all-important homeostasis needed for life: control of body temperature, blood pressure, fluid volumes, cellular concentrations of ions such as sodium, potassium, calcium, iron, etc, and metabolic substrates such as glucose. We also have a very good understanding of the physical conservation laws these physiological processes must obey: the conservation of mass, charge and energy, respectively. And these physical laws are just as important as the genetic code in explaining how our bodies work. Fortunately, the computing power needed to solve the equations arising from these physical laws is also now available.
So, where is the bioengineering community up to with creating human digital twins, and what in particular are we at the Auckland Bioengineering Institute (ABI) doing to facilitate their development and application to healthcare?
Given the fact that nearly all drugs only work on 50 percent of the population, there is an opportunity to use a diverse population of personalised digital twins for testing drug efficacy with virtual clinical trials
Just as experimental results must be repeatable and use documented experimental protocols to be of value to science, mathematical models must be reproducible and validated against experimental data. These models must also be well documented and annotated for reusability. The ABI has led the international Physiome Project for over 20 years, creating modelling standards, a model repository, software tools and an open access journal for physiological modelling.
Together with colleagues around the world, the ABI has also established a mathematical framework for modelling the anatomy of the body and for assembling the vast array of biophysical mechanisms underpinning physiology. The success of the human digital twin will depend on a coordinated international effort to encapsulate as much physiological detail as possible within this modelling framework over the next few years.
Today, the predictions of the complex physics-based models can also be used with machine learning or AI to train less computationally expensive surrogate digital twin models for clinical applications. To assist with these efforts, the NZ Government’s Ministry of Business, Innovation and Employment has funded a Catalyst project at the ABI, 12 Labours (after the 12 organ systems of the body).
Although a comprehensive model of the entire human body that can be personalised and used for diagnosis and treatment planning is many years away, there are many shorter-term clinical outcomes that can benefit from the digital twin approach.
Heart models, for example, are currently routinely fitted to patient MRI and ultrasound data for assessing regional cardiac muscle function and the dependence of diseases such as cardiac hypertrophy on the function of particular proteins is being elucidated with these models. The Food and Drug Administration in the US has recently accepted the use of mathematical modelling as part of the approval process for a drug.
Researchers in the ABI are developing biophysically based models of tissues and organs for most of the body’s organ systems. The models usually target specific clinical goals but as these are brought into the common mathematical infrastructure for the digital twin, the models contribute to our larger scale understanding of integrated whole-body physiological systems.
Given the fact that nearly all drugs only work on 50 percent of the population, there is an opportunity to use a diverse population of personalised digital twins for testing drug efficacy with virtual clinical trials – and of course using their digital twin to find the appropriate combination of drugs that work for that person. We are a long way from reaching this aspiration, but it is a feasible goal.
A key aspect of a human digital twin is that, like the aircraft engine mentioned above, it is personalised as much as possible to an individual and continually updated with new data as new measurements are performed on that individual. Often the initial creation of the personalised model requires the use of expensive hospital imaging equipment but once the personal digital twin has been created, the parameters of the model can be updated based on data from wearable, or in some cases implantable, devices that can provide continuous data with minimal need for clinician time and hospital appointments.
The concept of ‘digital twins’ for engineering systems has been around for many years, keeping us safe as we fly around the globe, and the same principles can be applied to maintaining, understanding and supporting the health of the human body.
The Auckland Bioengineering Institute is hosting Bioengineering the Future, a week-long free public event showcasing research that aims to enhance diagnosis and treatment of a range of medical conditions. Find out more at Eventbrite.