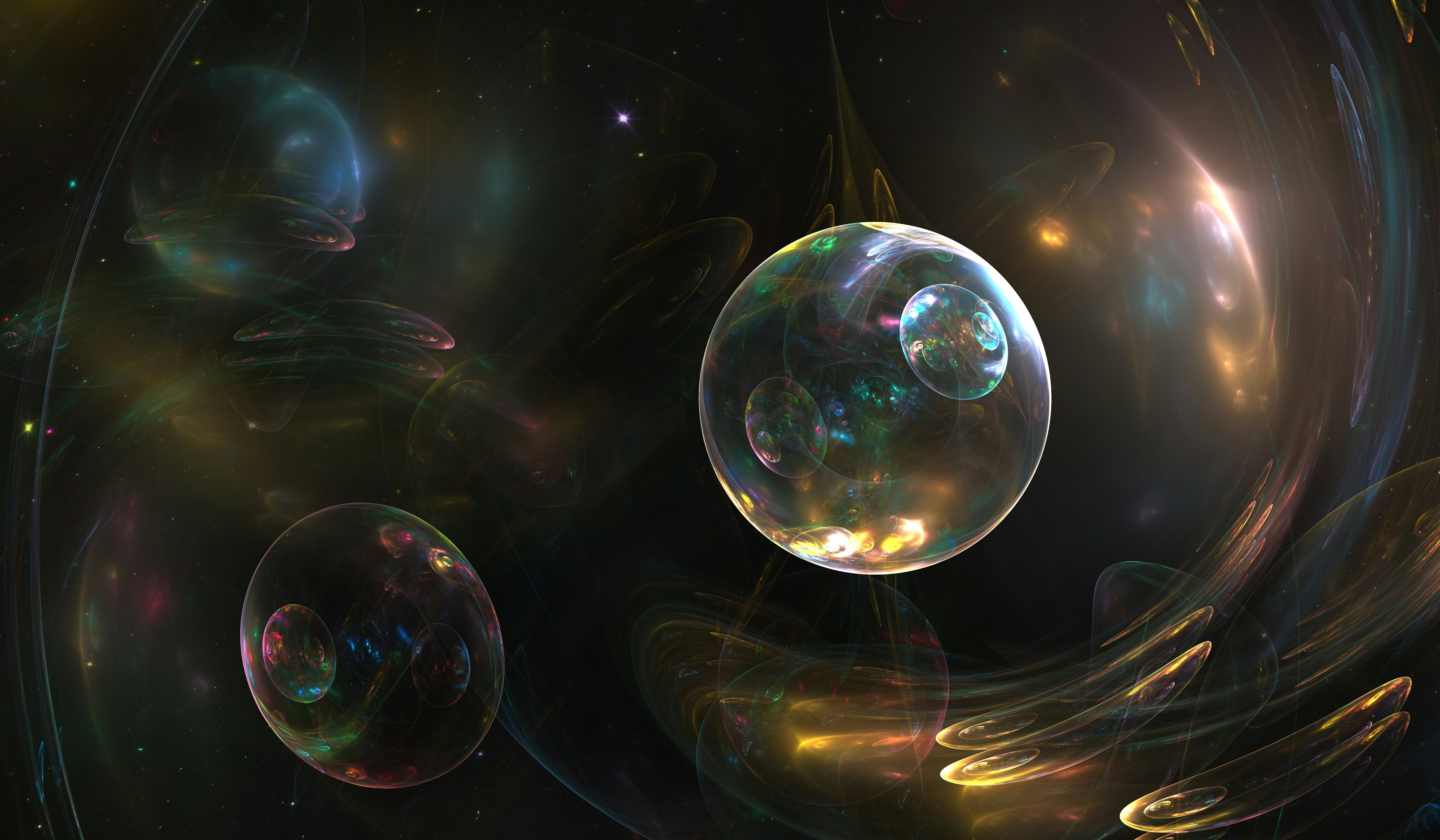
A physics experiment turned up no signs that the elusive particles called Majorana neutrinos exist, according to a new study published in Nature today, but that doesn’t mean it won’t lead to new discoveries.
The Cryogenic Underground Laboratory for Rare Events (CUORE) collaboration published its latest findings on the hunt for a debated property of neutrinos — particles so light and rare to interact with other matter that they’re often nicknamed ghost particles. This property, if present in the fundamental particles, could explain why the universe has any matter left at all from the Big Bang.
Scientists still don’t know some of the fundamental properties of neutrinos, like how massive they are, so finding this out “would change the way we model the evolution of the universe,” because neutrinos are the most abundant massive particle in the universe, Frank Avignone, a particle astrophysicist at from University of South Carolina who has been part of the CUORE project since the beginning, tells Inverse.
Here’s the background — CUORE consists of 988 detectors, each made of a 2-inch-wide cube of tellurium dioxide crystal with an incredibly sensitive thermometer attached, built into towers and placed deep underground at the Gran Sasso massif in Italy. The cubes are kept at super-low temperature, operating at 11 to 15 millikelvin (thousandths of a degree above absolute zero). The cubes are sensitive enough to see the small changes in temperature caused by radioactive decay.
The idea of the network of tiny detectors came from Ettore Fiorini at the University of Milan. “I once told Fiorini that he was crazy, turns out I was,” Avingone says. At the time Fiorini was experimenting with detectors the size of sugar cubes, while Avignone was using ones that weighed several pounds.
One of the major mysteries that the collaboration seeks to shed light on is the balance of matter and antimatter in the universe. During the Big Bang as many particles were created as antiparticles. But as the universe started to cool the particles and antiparticles started to join up and annihilate each other, Avignone says. So, why didn’t the matter and antimatter destroy each other completely, or leave both types around? After all, “we don't see lots of anti-protons … no matter where we look,” he says.
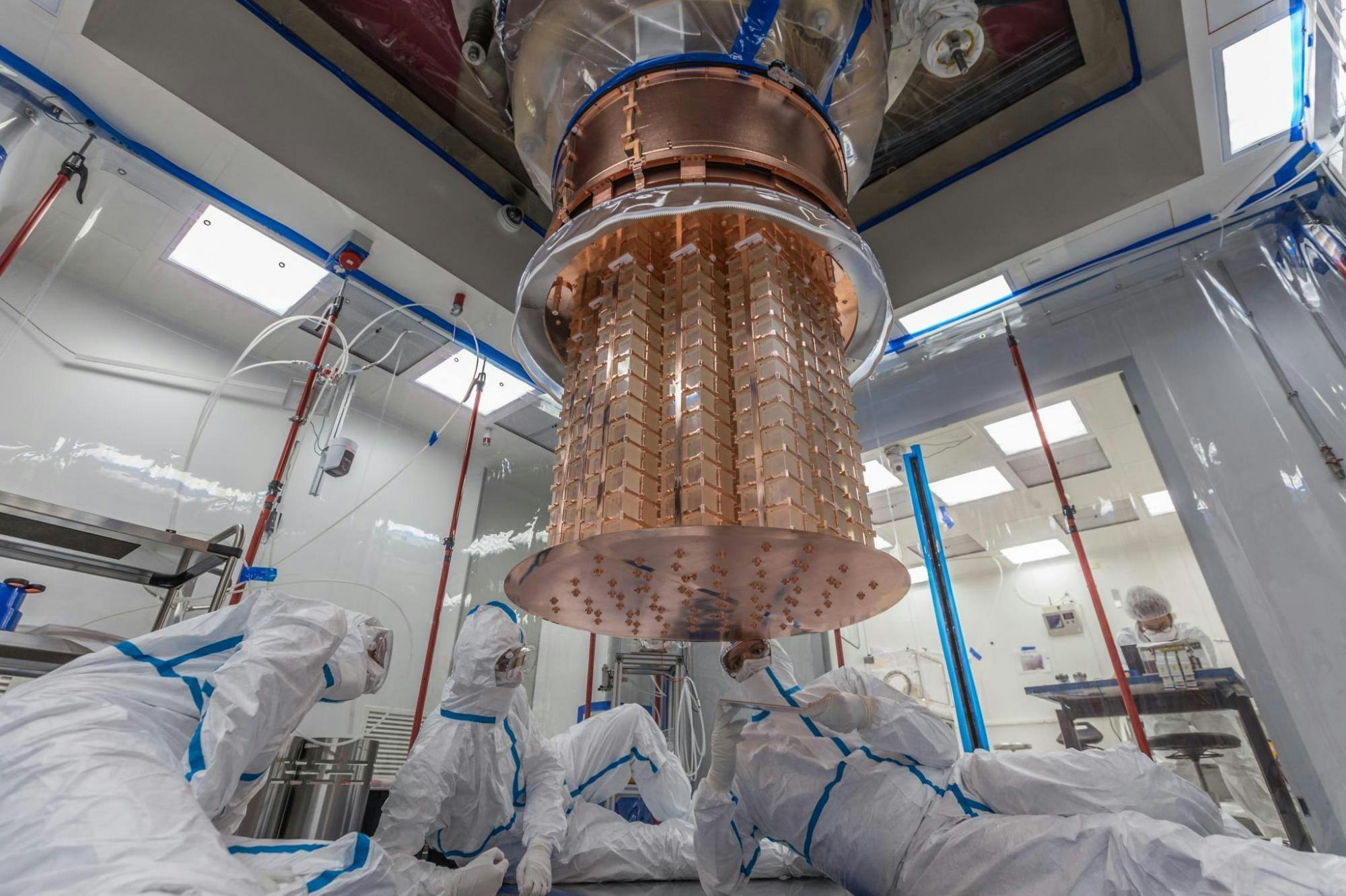
What they did — The most popular explanation as to how this happened is called leptogenesis and it requires that neutrinos are Majorana particles, which essentially means they are their own antiparticle and when two collide they would self-annihilate—destroying each other and leaving only energy.
Closely watching a type of radioactive decay is “the only practical way” to find out if the neutrino is a Majorana particle, Avignone says. In a normal beta decay, a neutron decays into a proton, electron, and neutrino. In a double beta decay, that process happens twice, “in a chain,” he says.
If they’re Majorana particles, the two neutrinos produced in rapid succession in this double beta decay would be able to recombine and vanish, leaving only energy. If they aren’t, both neutrinos would survive.
Neutrinos interact with matter so rarely that picking up any two is next to impossible, but in these crystal detectors scientists can also study these radioactive decays through the total energy that the sensor picks up during a decay. That amount of energy will change depending on whether neutrinos escape.
What’s new — The team chose the isotope tellurium-130 for their detector cubes because it will naturally undergo a double beta decay, transforming first iodine, then xenon as it emits electrons and neutrinos.
If the neutrinos disappear, the decay would produce a spike of energy at a specific frequency. This data would provide evidence that the neutrinos are Majorana particles and help determine the neutrino’s mass.
If neutrinos are emitted however, they will affect the energy release d— turning it into a broader “continuum” of frequencies for the sensor to pick up, as opposed to one frequency, Avignone says.
In the team’s newly published results they see no sign of the energy spike that would indicate neutrinos are Majorana particles, but that doesn’t mean the spikes don’t exist—the team has just shown that the spike can’t be detected with this sensitivity of their instrument. Which is why the collaboration is already planning an upgrade called CUPID that would use a different crystal to detect two signals—a heat signal and a light signal from the radioactive decay that could be combined to get even better sensitivity.
The concept of CUPID has got early approval from the Department of Energy, but it still has a long way to go before it’s realized, Avignone says. In the meantime, CUORE will keep operating, racking up detections to hunt for signs of Majorana neutrinos.
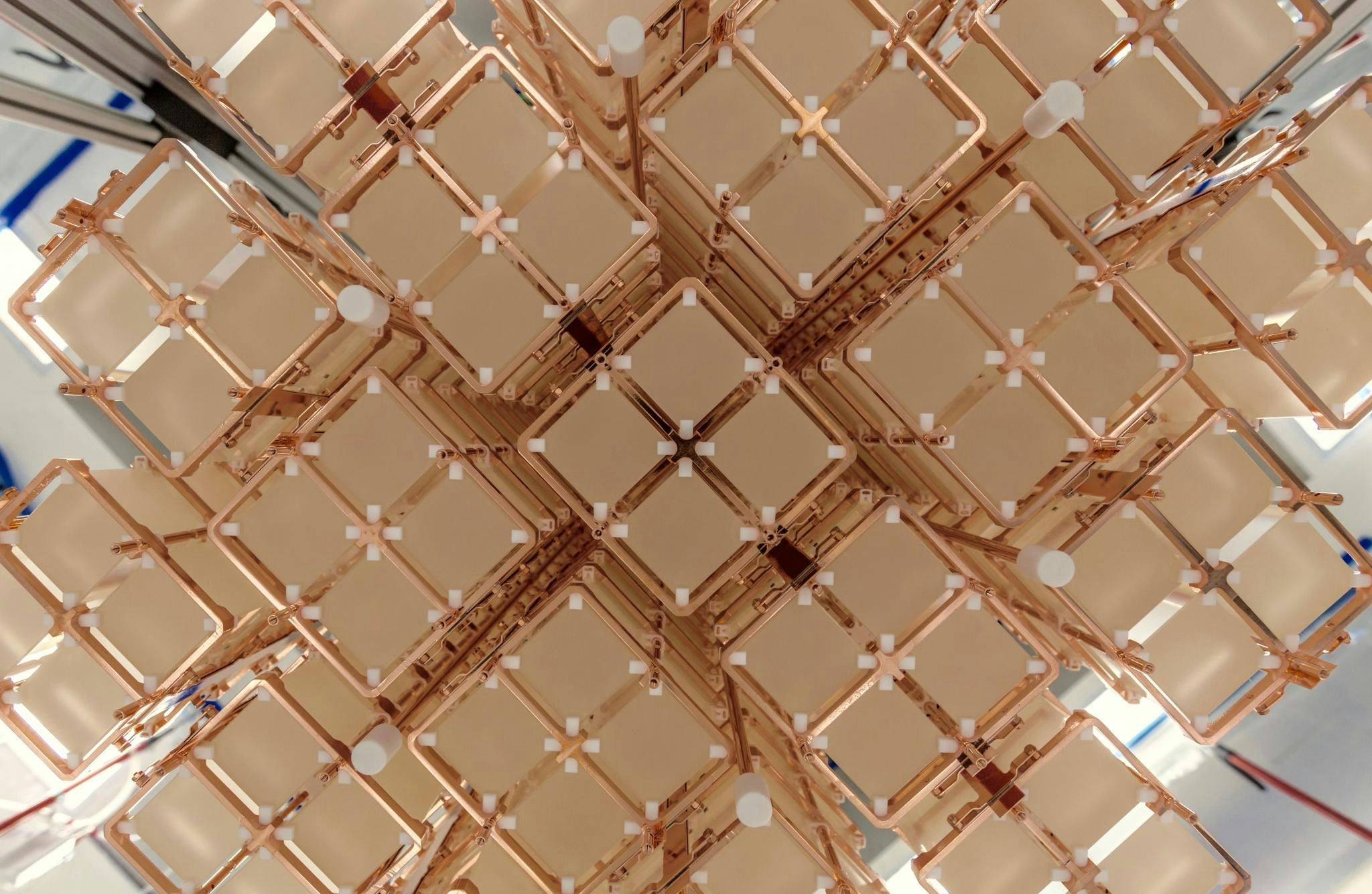
Why it matters — Not finding the particle doesn’t mean the experiment has been a failure. The measurements have helped narrow down the properties of the neutrino — indirectly getting a better ballpark of its mass, he says.
Establishing the boundaries on what the particle’s mass can be is important for cosmology — understanding the big-picture structure and formation of the universe. That’s very valuable data, Avignone says, “even if we never see it.”
In a presentation, he once dubbed the project “the search for neutrino properties hiding under an Italian mountain.”
People sometimes ask him why the experiment is in caverns beneath an Italian mountain. He replies that the layer of solid rock shields from the ever-present cosmic rays which would interfere with the experiment, Avignone says. Also, he says, “the wine is good there.”