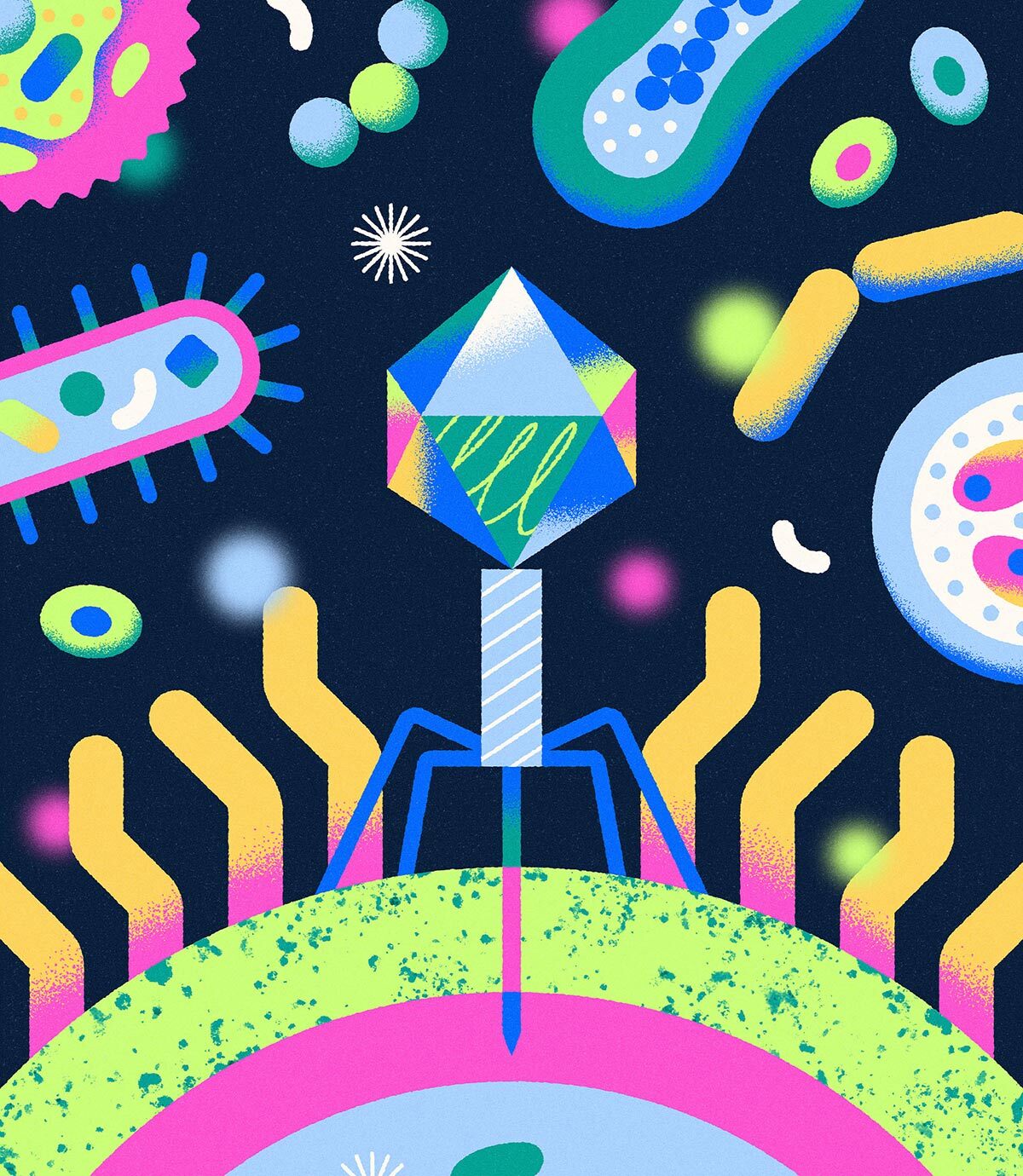
Infectious diseases physician Stephen Vaughan stands at a sink in Calgary’s South Health Campus wearing a surgeon’s scrubs, cap, and mask, washing up with orthopaedic surgeon Stephen Hunt. Inside the operating room, the two men receive sterile towels and gowns and take their place next to seventy-four-year-old Boyd English, already asleep on the table. English has a hip infection and he needs to have the joint washed out. Again.
Hunt draws a scalpel across English’s right hip. None of the three men can remember how many surgeries English has had, but according to Hunt, when it comes to English’s hip, “everything [has been] taken out and put back in. His X-rays give an orthopaedic surgeon shivers.” To the steady beep of the heart monitor, Hunt dissects down to the cold steel glint of the prosthetic English has as a result of an accident on an oil rig in the Yukon in 1973 that sent him crashing to the ground, breaking part of his femur. Infection set in something like forty years ago. Recently, fluid has frequently been draining to the outside of his body, soaking through dressings and even his pants until another surgery or round of antibiotics knocks back the infection. Today the two Steves are going in, for what they hope will be the last time, to clean out the joint and take samples of English’s infected tissues. (Hunt is my husband, and we both know Vaughan from medical school.) The samples will be sent to the lab to identify which bacteria are growing and which antibiotics might work.
This kind of washout is done nearly every day in orthopaedic surgery. But there’s a twist to this one, and that’s why Vaughan’s here today. English is fed up with a lifetime of antibiotics. He’s had a series of side effects—some life threatening—from the long list of those he’s tried in the past, which, in combination with other treatment expenses, cost him about $1,000 per week. And he’s tired of the pain.
Vaughan wants to try a different therapy, one that makes use of millions of years of microbial evolution and employs naturally occurring bacteriophages to kill off the infection. Though it’s been around for a hundred years, phage therapy is still considered experimental, and Vaughan will have to apply to Health Canada to run a clinical trial with English as the single patient. Before that, he’ll have to find a research lab that has the specific bacteriophages that will work on English’s bacteria. And even before that, he’ll have to get just the right sample of tissue containing the bacteria. That’s today’s job. Hunt removes tissue and chisels out bits of English’s femur, sewing his ravaged tissues back together as best he can. Vaughan strips off his protective gear and leaves the operating room to hand-deliver his precious cargo to the lab.
BACTERIOPHAGES, or phages for short, are viruses that attack bacteria and kill them; the two organisms have been involved in an evolutionary cat-and-mouse game for millions of years. Phages are ever present in the environment, from sea water to barnyard waste—anywhere bacteria are found in high numbers.
Phages were first discovered in 1917 by a French Canadian scientist named Félix d’Hérelle and were soon used to treat humans. In 1935, H. Gurth Pretty, a surgeon at Montreal General Hospital, gushed in the pages of Canadian Medical Association Journal that the discovery of bacteriophages had “entirely revolutionized the treatment of carbuncles, furuncles and allied superficial infections of the body.” (Furuncle is another word for “boil,” and a carbuncle is a cluster of boils.) Phage cocktails were administered orally—“one ampoule in a wineglass of Vichy water”—or by injection under the skin over three successive days, requiring just a few millilitres of bacteriophage for a carbuncle “the size of an English penny.” By the mid-1930s, bacteriophage cocktails were available for several bacteria responsible for virulent gastrointestinal infections, as well as for Staphylococcus aureus, a bacterium frequently implicated in skin infections. Pretty ended his paper with a thank-you to the Anglo-French Drug Company “for an unlimited supply of bacteriophage.”
But bacteriophages are often highly specific to one strain of bacteria, and it takes time to grow the bacteria in vitro and hunt down the right phages. And finding a match is not the end of it. Phage cocktails need to be highly purified so that patients receiving them are not at risk of going into shock. Then, researchers have to make sure the dose of phages is correct. An acutely ill person can’t always wait for all of that to happen because infectious diseases can move quickly. “Infected patients either get treated and get better, or they die,” Vaughan says. “It’s us versus the bacteria, and they can evolve a lot quicker than we can.”
All of which made antibiotics, widely available since the first clinical administration of penicillin in 1941, quite attractive. Antibiotics generally work across many strains of bacteria, and they work quickly. Many scholars and physicians consider them the greatest medical breakthrough of the twentieth century. Antibiotics made previously lethal infections such as tuberculosis and pneumonia treatable and contributed to an increase of more than twenty years in life expectancy in the United States.
But while antibiotics have saved millions of lives around the world, their liberal use globally—as well as a lack of access to clean water and sanitation in much of the world—has led to terrifying rates of antimicrobial resistance. Research in The Lancet suggests antimicrobial resistance was associated with the deaths of nearly 5 million people in 2019 and that this number could double by 2050, nearly catching up to the number of deaths caused by cancer in 2020.
Here’s how it happens.
As living organisms, bacteria are encoded by DNA, and DNA occasionally mutates. Sometimes genetic mutations render a bacterium immune to an antibiotic’s chemical tactics. The few cells that might escape antibiotic pressure then have a sudden advantage: with their counterparts wiped out, resources abound, and the remaining antibiotic-resistant bacteria proliferate. It’s a problem not only for the host—you or me when we are treated with an antibiotic and develop a resistant strain—but also for anyone with whom we happen to share our resistant bacteria, say, on a door handle or keyboard. In fact, most resistant bacteria develop not in people but in livestock fed antibiotics to promote growth; these resistant bacteria infect people through contaminated animal products. This is how even antibiotic “naive” people come to be infected with resistant strains of bacteria.
I see this all the time as a family doctor. A woman has a urinary tract infection. I tell her that her bacteria are resistant to this or that antibiotic, and she says, “But I’ve never taken any of those.” Welcome to the global human soup.
It’s a conversation Vaughan, too, often has with patients, and it worries him. “I think it’s very likely in our life we’ll be at a point where there might only be one or two antibiotics to treat some infections, and there will be some pan-drug-resistant organisms,” he says. “Those have already been reported in the literature. I think we are in trouble.”
While phage therapy was largely abandoned after the 1940s during the so-called golden age of antibiotics, microbiologists in Eastern Europe and former Soviet republics continued using phages in research and clinical applications. Only since 2017 have researchers in the US started applying to the Food and Drug Administration’s emergency Investigational New Drug (eIND) program for permission to try phage therapy for conditions such as cystic fibrosis–related lung infections, infected burns, bacterial diarrhea, and Staphylococcus aureus bloodstream infections. A highly publicized story about phage therapy being used to treat a life-threatening infection can be found in Steffanie Strathdee’s 2020 memoir, co-authored with Thomas Patterson, The Perfect Predator: A Scientist’s Race to Save Her Husband from a Deadly Superbug. Some things about present-day phage therapy resemble the early days of treatment, with preparations for oral administration and injection into sites of infections. But in the past decade, intravenous preparations and improved purification methods have also been developed. Research labs have also established large libraries of phages so that strains no longer have to be sought in the wilds of sewage treatment plants and barnyards, though these libraries require continual expansion.
In 2023, Greg German, a medical microbiologist at Unity Health Toronto, treated a seventy-two-year-old woman for a debilitating urinary tract infection she had spent years trying to treat with antibiotics and to which she had lost a kidney. After phage therapy, German says, the patient began to feel better within two days. Her treatment eventually involved a combination of phages and antibiotics, but a month after she began with the phages, she reported feeling better than she had in about seven years. Prior to that, only one other person had been treated in Canada in modern times: a youth with a cystic fibrosis–related lung infection in 2022.
Almost immediately after media reports of German’s successful treatment, he started receiving emails and tweets from people hoping to try phage therapy and have it supervised by a Canadian physician. Recently, the University of Toronto, where German is an assistant professor, received an anonymous gift of $5 million to accelerate phage therapy and innovation in Canada. He envisions a time within the next fifteen years when scientists will be able to design phages specific to a patient’s bacterial strains rather than having to look for pre-existing matches.
German has collaborated at times with Nancy Tawil, a biomedical engineer with experience in phage production for the US-based company Precisio Biotix Therapeutics and as CEO of Qeen BioTherapeutics based in Gatineau, Quebec. For years, her production teams have been collecting libraries of phages from all over the world that infect and kill common human pathogens, such as Staphylococcus, Pseudomonas, Klebsiella, and Acinetobacter, all of which can cause life-threatening lung, brain, and blood infections. To date, most of Tawil’s research has been done on patients in the US. Qeen receives a sample of infected tissue, isolates the bacteria, screens its library for an effective phage—usually a cocktail of up to five phages—then ferments, multiplies, and purifies the phages, and delivers the mixture to the clinical team. That process takes between a week and two weeks. The price tag? About $45,000. According to Tawil, that’s the cost of making 200 litres of phage solution, whereas a patient might need only fifty millilitres for their treatment. Sometimes a phage found for one person can also work on another, reducing the cost of treatment per person.
Tawil has done some pro bono work for Canadian researchers, but that can’t go on forever, especially when the US is so much more amenable to getting phages to the bedside and the market. Doctors there can get approval to treat patients in a matter of days. “Just getting a sit-down with Health Canada is difficult,” Tawil says, though she feels the process is slowly becoming smoother.
She’s hopeful one of the first patients to be approved by Health Canada will be Boyd English. After German’s successful treatment in 2023, he put Vaughan in touch with Tawil, and she’s already found them a match.
On a Sunday afternoon in April 2023, I visit English in his home outside of Airdrie, Alberta. Gravel roads and open fields stretch for miles around. We sit in the living room of his ranch-style house, English in an easy chair, scratching his collie, Bee, on the chin, his crutches propped up beside him. He tells me about the accident fifty years ago and how he waited hours for medical care after falling from the rig in Old Crow, Yukon, which wasn’t accessible by road. He had to be flown to Inuvik and then to Calgary, where he had surgery and a protracted hospital stay, losing seventy pounds and feeling depressed. Rehab didn’t go so well. His marriage ended. He went back and forth between living in his car and staying in cheap hotels.
Gradually, he got back on his feet and started his own business, remarried. In 1981, the hardware in his femur loosened, and he needed revision surgery. Then, a life-threatening reaction to the antibiotic vancomycin landed him in the hospital. He eventually met Vaughan, who has treated him for the past decade.
The definitive cure for English’s chronic pain and infection would be amputating his leg and part of his pelvis. Faced with that, English would rather die—he’s specified this in his advanced health care directive. Phage therapy is an alternative, and English could become the first in Canada to receive it for an infected prosthetic joint. Around 100,000 hip and knee replacements were done in Canada in 2020/21; a 2017 study put the rate of post-operative infection at 1.6 and 1.5 per 100 procedures of hip and knee replacements respectively. Most are treated much more easily than English’s, but he hopes that even if he doesn’t benefit from phage therapy directly, what Vaughan learns in the process will help others avoid what he’s had to live through over the past half century.
But it’s taking a lot of patience. When I spoke to Vaughan in April 2023, a few days before he collected the samples from English, he figured it would take six months to find the right phage cocktail and receive ethical approval to administer it. He wasn’t sure how he would pay for it. “Boyd has aged a lot as a result of this,” Vaughan said back then. “He’s a lot more frail than he was when I first met him. His strength is down.” Today, with a match in hand, Vaughan is waiting for approval from Health Canada and remains hopeful the treatment will eventually work.
English, for his part, is no stranger to patience. The summer and fall came and went with more drainage, more antibiotics, more side effects and weight loss. In February, he was still waiting.
Correction, March 18, 2024: An earlier version of this article misspelled author Steffanie Strathdee’s first name. The Walrus regrets the error.