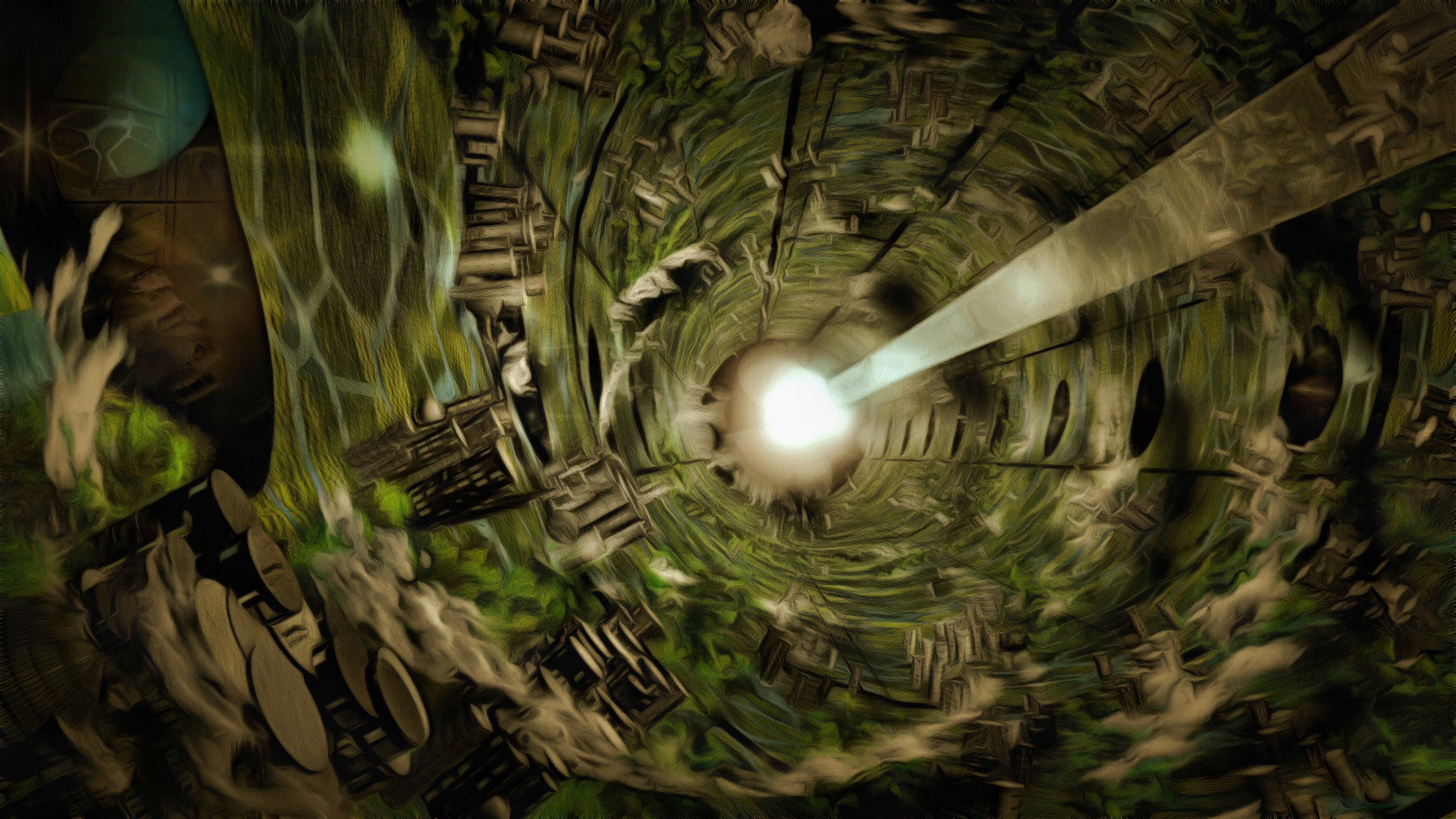
During the 1960s and 70s, coinciding with the height of the Space Age, scientists pondered how human beings could one day live in space. Among the many benefits, the migration of humans and industry to other celestial bodies and orbiting habitats presented a possible solution to overpopulation and environmental degradation. As Gerard K. O’Neill suggested in his writings, the key was to make this migration an economically feasible venture. Given the renewed efforts to explore space that are now underway and the rise of commercial space (NewSpace), there is a growing sense that humanity’s migration to space is within reach — and even inevitable.
But to paraphrase famed British historian AJP Taylor, “nothing is inevitable until it happens.” In a new study, Cornell graduate researcher Morgan A. Irons and Norfolk Institute co-founder and executive director Lee G. Irons reviewed a century of scientific studies to develop the Pancosmorio (“World Limit”) theory. They concluded that specific life-sustaining conditions on Earth that are available nowhere else in the Solar System could be the very thing that inhibits our expansion into space. Without an Earth-like “self-restoring order, capacity, and organization,” they argue, space settlements would fail to be sustainable and collapse before long.
Lee G. Irons is the founder of the Norfolk Institute in Virginia, a firm specializing in solutions for living in space and sustainably here on Earth. Morgan A. Irons is a Ph.D. candidate, a member of Johannes Lehmann’s research group at Cornell University, and a Carl Sagan Institute Research Fellow. In 2016, Morgan and Lee founded the Virginia-based startup Deep Space Ecology (DSE) on engineering and designing ecological systems to provide food sustainably in space and on Earth. In 2020, Morgan and Dr. Lehmann at Cornell University, with the support of Norfolk Institute, DSE, the Zwillenberg-Tietz Foundation, bio365 LLC, Rhodium Scientific LLC, and Dr. Matthias Rillig at Freie Universität Berlin, sent a soil experiment to the ISS that measured the gravitational effects on soil stability – a.k.a. the “Soil Health in Space” experiment.
Migration and Earth
The paper that describes their research recently appeared in the Frontiers in Astronomy and Space Science. As a primary consideration, Lee and Morgan Irons addressed the assumption behind the belief that humanity’s migration to space is inevitable. As is often the case, proponents of this theory draw parallels between this supposed future and humanity’s long history of migration. These migrations saw humanity travel from Africa to Eurasia, Eurasia to Australasia and Polynesia, and Asia to the Americas, all within 200,000 years.
Linguists and ethnographers have also traced multiple migrations over the past 15,000 years that profoundly affected history, modern language, and culture. These include the Proto-Indo-European (PIE) migrations throughout Eurasia during the Neolithic period (ca. 10,000 to 5,000 years ago), the migrations of the Austronesian peoples across the South Pacific (ca. 3000 BCE to 1000 CE), the Bantu expansion across Africa (ca. 1000 to 500 BCE), the “Barbarian Invasions” of Europe (ca. 300 to 800 CE), and the European colonization of the Americas, Africa, and Asia between the 16th and 20th century CE.
And who can forget the immortal words of Carl Sagan, “Exploration is in our nature. We began as wanderers, and we are wanderers still. We have lingered long enough on the shores of the cosmic ocean. We are ready at last to set sail for the stars.” Another gem is the passage he wrote in “Pale Blue Dot: A Vision of the Human Future in Space,” which was featured in the short film “Wanderers” (see below). As Sagan expressed:
“For all its material advantages, the sedentary life has left us edgy, unfulfilled. Even after 400 generations in villages and cities, we haven’t forgotten. The open road still softly calls, like a nearly forgotten song of childhood. We invest in far-off places with a certain romance. This appeal, I suspect, has been meticulously crafted by natural selection as an essential element in our survival.
“Long summers, mild winters, rich harvests, plentiful game—none of them lasts forever. It is beyond our powers to predict the future. Catastrophic events have a way of sneaking up on us, of catching us unaware. Your own life, or your band’s, or even your species’ might be owed to a restless few—drawn, by a craving they can hardly articulate or understand, to undiscovered lands and new worlds.”
These words also articulate another critical aspect of this idea: how it is essential to survival. Many scientists and advocates for space migration, from Stephen Hawking and Robert Zubrin to Elon Musk, have emphasized that becoming “interplanetary” is the only long-term defense against extinction. Whether the cause is anthropogenic (climate change, nuclear war, etc.) or due to an asteroid impact, a gamma-ray burst, or some other cosmic phenomenon, being bound to one location leaves human civilization vulnerable.
As Sagan also noted, one look at our biochemistry confirms that all of the elements fused in the interior of stars are part of our makeup. From the water in our tissues, the nitrogen in our DNA, the calcium in our teeth and bones, the iron in our blood, and the elements (carbon, hydrogen, and oxygen) that are the building blocks of the proteins and lipids that make up our muscles and organs. As Sagan summarized, “we are made of star-stuff.” At the same time, we are bound to planet Earth through roughly 4 billion years of species evolution.
As stated by Lee and Morgan in the Pancosmorio theory paper, this evolutionary process led to the complicated ecological systems that sustain all life here on Earth. In this system, the energy contained by the interactions between all matter and the gravity field of Earth and the electrical fields of atoms is conserved – no irreversible entropy occurs. As a result, the ecological system is regenerative, replenishing itself over time to ensure stability and sustainability. The question then is, what are the consequences of humanity leaving Earth and its life-sustaining regenerative systems behind? And, more importantly, how will this impose limits on human growth beyond Earth?
A Big Ol’ Heat Pump
To address these questions, Morgan and Lee Irons developed the Pancosmorio theory based on ecological thermodynamics theory and the methodology of abductive reasoning. In this sense, the Earth and its regenerative ecosphere are similar to a self-restoring heat engine, where solar radiation is used by lifeforms and the abiotic environment to create energy, organic molecules, and biomass. Therefore, this comparison provides a basis for measuring sustainable growth.
Their proposed method also incorporates the basal growth hypothesis, which infers that activity in any location has a self-restoring order, capacity, and organization equivalent to the base of the ecosphere in which life evolved. As Lee Irons explained to Universe Today, their theory establishes:
“[T]hat human life and all Earth life evolved from ongoing solar power pouring down on Earth and a build-up of exergy (stored energy) in the form of networks of dissipative structures (biochemical cycles of lifeforms) that the life is now dependent on. The theory is also based on modeling these dissipative structures as well as those of geophysical cycles (e.g., water, air, and weather cycles) as heat engines and applying what we know in classical mechanics of conservative forces that make these heat engines self-restoring.”
In short, life on Earth evolved under certain conditions that are required to sustain human life at its current level of growth. Therefore, the availability of these conditions will define the limits of humanity’s growth in space. Integral to this is the existence of a self-restoring order that comes down to quantifiable factors (gravity, air pressure, heating, seasons, tidal forces, and radiation protection), robust networks of abiotic and biotic (inorganic and organic) resources that can endure stress loads, and a sufficient amount of land area to capture and distribute exergy.
Then, they considered how human infrastructure and technology could augment a basal system equivalent to what we’ve done here on Earth. This consideration essentially states that humans will need infrastructure comparable to what we have established here on Earth to maintain our current level of growth. This would require the in-situ resource utilization (ISRU) of the basal ecosphere, augmented power systems, agricultural production, and manufacturing capabilities that rival Earth’s. Said Lee:
“The nature of non-self-restoring augmentation systems (i.e., human technology, infrastructure, society, and civilization) that utilize irreversible cycles is that they require self-restoring basal systems (i.e., the naturally evolved ecosystems) that utilize semi-reversible cycles to be sustained. This is known as ‘strong sustainability.’
“It all comes down to the availability of stored exergy that contemporary humans have become adapted to on Earth,” said Lee. “Humans can certainly adapt to a lower exergy level, but that means that human augmentation capacity and organization would reduce in some way, likely uncontrollable. And going through that kind of adaptation in space without the buffering of Earth or someplace like Earth to back you up is very risky.”
This work builds on previous research by Morgan and Lee that showed how traditional Environmental Control and Life-Support Systems (ECLSS) used for short-duration missions in space could not sustain long-duration missions to the Moon, Mars, and beyond. For this, a Bioregenerative Life Support System (BLSS) that can replenish itself over time would be required. A key part of this study was the Terraform Sustainability Assessment Framework (TSAF) developed by Morgan and Lee to measure the effectiveness of a BLSS.
In this framework, a system that effectively mimics Earth’s regenerative capacity and resiliency would be ranked as a 1. In other words, if you can engineer a regenerative system in space that is at least as sustainable as a similar system on Earth, then you’ve effectively formed an Earth-like system in space (aka. “terraformed”). Lee and Morgan have further developed this framework into a quantitative method of human sustainability as part of the Pancosmorio theory.
Four Levels
Based on these conditions, Morgan and Lee then evaluated how humans could live beyond Earth using four analytical models. Based on the previous considerations, these models incorporate different degrees of self-sustainability and dependency on Earth. As they demonstrate, this directly impacts how far settlements can be established from Earth and imposes strict limits on their growth.
The four models are arranged hierarchically into four levels, where Level 1 applies to habitats and settlements that have achieved terraform-specific stability ratings of 1, while Levels 2 to 4 have lower ratings. Here’s how they break down:
Level 1: Space habitats or settlements with sufficient dissipative structures, power, area, functional diversity, network vitality, and sufficient infrastructure to augment the basal ecosystem with contemporary human society. This refers to an advanced human society in space that has effectively solved the issue of human sustainability and does not require supply chains with Earth. This is the recommended level of development for human bases and settlements located well beyond the Earth-Moon System.
Level 2: Habitats and settlements with a sufficient self-restoring order and capacity with dissipative structures, power, and area conditions being met. However, these habitats have insufficient organization, either with the ecosystem or the infrastructure, resulting in a brittle network subject to blight and diversity loss when disturbed. This level allows for innovation and adaptation but requires a minimal supply chain to replace deployed resources and specialized technology.
Level 3: Self-restoring order is established, but the capacity is not met, resulting from insufficient power and/or area to ensure human adaptability. This results in sufficient human life support but an insufficient level of organization to mitigate a cascade failure in the event of disturbances. This is only recommended as an early stage for human habitats or settlement and requires significant improvements while supported by a supplemental supply chain.
Level 4: The default when none of the sustainability conditions are met. For these habitats or settlements, self-restoring order, capacity, and organization are insufficient, and augmentation only provides mission operations systems and ECLS system. A. supply chain is absolutely necessary. This is recommended only for crewed missions within Earth orbit – like the International Space Station (ISS) – and uncrewed missions of any duration beyond LEO.
“Levels of sustainability effectively highlight how much risk the humans of the settlement are under,” Lee summarized. “The problem is that deep space locations are not going to be able to rely on supply chain support from Earth because supply chains from Earth themselves are not going to be reliable or sustainable until they are as ubiquitous as the supply chain system on Earth. So, human sustainability in space is fundamentally about a settlement’s ability to sustain its local human population when supply chains from Earth are not available.”
Five Hypothesis
Finally, Morgan and Lee subject their four sustainability models to five testable hypotheses that utilize their new quantitative method of human sustainability. Each of these comes down to the results of controlled tests or human missions, as bases and settlements migrate out into the Solar System and experience unplanned events – from the loss of productivity and blight to diversity loss and cascade failure. The five hypotheses and their respective tests are organized as follows:
The Growth Hypothesis: Any human base or settlement in space operating at Level 1 sustainability can experience growth that can sustain all planned loads without disruption and adapt to unplanned loads and disruptions with a minimum of time. In short, this base would have terraform-specific stability ratings of 1.
Test: To determine the causal load or disruption, perform a root cause analysis of an unplanned event that results in a temporary drop in production of an ecosphere that meets Level 1 sustainability criteria.
The Blight Hypothesis: Any human base or settlement in space operating at Level 2 sustainability or lower can experience ecological or technological networks faltering under planned loads without any subsequent disruption. This lack of robustness would appear as an ecosystem blight and a limited loss of production, requiring corrective and preventative actions to restore production.
Test: Perform a root cause analysis of an unplanned event that results in a drop in production without recovery (aka. blight) in an ecosphere that meets Level 2 sustainability criteria.
The Diversity Loss Hypothesis: Any human base or settlement in space operating at Level 2 sustainability or lower can experience disruptions driven by planned loads and lack of full recovery from any disruption. This lack of reliability would appear as a limited loss of functional diversity, resulting in the local extinctions of species and the loss of technological capability.
Test: Perform a root cause analysis of an unplanned event that results in a loss of diversity without recovery of an ecosphere that meets Level 2 sustainability criteria.
The Cascade Failure Hypothesis: Any human base or settlement in space operating at Level 3 sustainability or lower can experience disruptions without recovery. This would appear as a cascading loss where a resource is fully depleted, causing a chain reaction that risks the total loss of other resources. The result would require the creation of an emergency supply chain from another location or abandoning the location to save lives.
Test: Perform a root cause analysis of an unplanned event that results in an actual cascade failure (a collapse with major and multiple losses) of an ecosphere that meets Level 3 sustainability criteria.
The Pancosmorio Theorem Hypothesis: There is a maximum number of astronomical units from the Sun out to which a terraformed system is sustainable (assuming it satisfies all Level 1 sustainability conditions).
Test: The hypothesis is validated by the failure of a base or settlement to provide sufficient power (solar, nuclear, batteries, power cells, etc.) based upon the bases’ or settlements’ distance from the Sun – which can then result in blight, diversity loss, or cascade failure. A base or settlement that meets the requisite conditions of dissipative structures and area but still has sustainability problems is evidence that it can’t achieve and maintain adequate organization due to inadequate capacity, either because of the distance from the Sun or insufficient power generation.
Some undeniable conclusions can be drawn from the Pancosmorio theory and its framework of four models, five testable hypotheses, and an abductive reasoning approach. First, human technology, infrastructure, and society cannot be sustained outside of Earth without having a sustainable basal ecosystem to sustain them. Second, life as we know it is a consequence of systems, feedback, and conditions unique to Earth. In other words, they exist nowhere else in our solar system, making establishing a human settlement outside of Earth much more difficult than simply building a dome, pumping up the atmospheric pressure, and spreading seeds and fertilizer everywhere.
Any base or settlement established beyond LEO in the near future (especially long-duration ones) will need to be as self-sufficient as possible. This includes having sufficient energy, dissipative structures, heating, gravity, the abiotic/biotic elements necessary to ensure order, and the right technology to augment these and fill in the gaps. Rotating cylinders, centrifuges, and nuclear reactors are good examples of how technology could provide simulated gravity and heating, but natural methods will also be needed.
The bottom line is that the farther a habitat or settlement is from Earth, the more self-sustaining it will need to be, but also, the more difficult it will be to make it self-sustaining. This means that the inhabitants will need to create an ecosphere that is as “Earth-like” as possible (i.e., they will have to create a “terraformed environment”) and find sufficient levels of sustainable power sources to be equivalent to the solar power received by Earth. Otherwise, these habitats or settlements must rely on expensive and unreliable supply chains or risk internal collapse. These limitations place strenuous limitations on how and where humanity can settle.
Implications
As with their previous study on BLSS, this research is significant since it establishes a quantifiable method for measuring sustainability. This could allow scientists and mission planners to calculate the likelihood of long-term success for missions destined for the Moon, Mars, and beyond. Wherever and whenever humanity plans to set up shop away from the comfort and life-sustaining system of Earth, these quantifiable frameworks will tell them exactly what they need to survive. Said Lee:
“Ecologists have been trying to work out how to quantify orthogonal sustainability properties for decades. We feel we have accomplished solving the orthogonal problem and the application of network ecology and ecological thermodynamics to space. We also feel we have theoretically figured out why and how Earth works functionally as an island of order and, therefore, what that means for living outside that island of order.”
Ultimately, the study provides three insights into humanity’s ongoing endeavors in space. The first concerns the International Space Exploration Coordination Group‘s (ISECG) plan to create a permanent human presence on the Moon. Based on their framework, Morgan and Lee state that this plan heavily depends on a very limited supply-chain support system and contains no backup augmentation reserve elements. Moreover, the emergency egress plans are no better than those of the ISS, which is completely impractical. In essence, the plan is no more sustainable than those governing a space station in LEO.
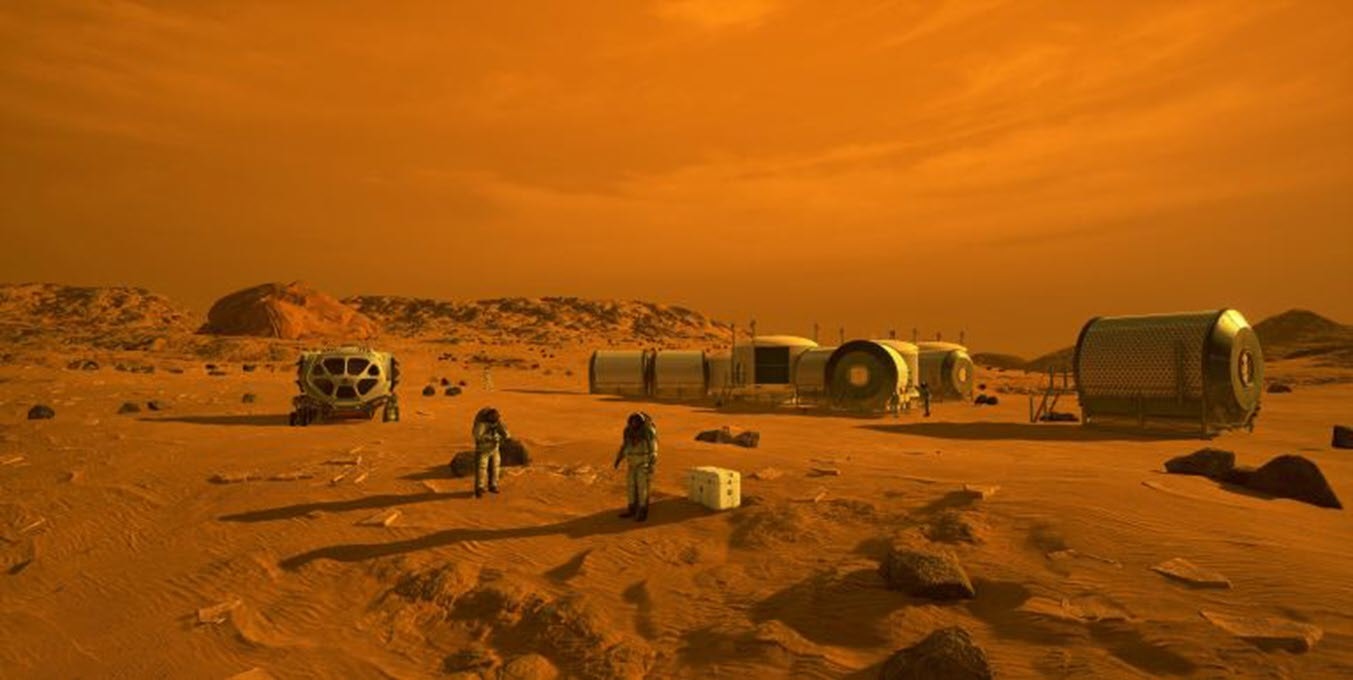
Second, this study has implications for creating a human settlement on Mars, which would have difficulty achieving any sustainability level. The distance from Earth rules out Level 4 sustainability, while Level 3 and above are challenging due to low gravity and gradient air pressure conditions. As they indicate, a settlement would need to simulate 1-g gravity to ensure that an ecosphere could survive long-term (which is difficult to imagine).
The third insight is perhaps the most intriguing and relates to our search for intelligent life elsewhere in the Universe (aka. SETI). Given that the laws of physics are universal (and with the Copernican Principle in mind), it is safe to say that this Pancosmorio theory can apply to any life that evolved anywhere in the Universe. As Lee explained, this presents a possible resolution to the Fermi Paradox:
“The theory suggests that other life forms in the universe likely evolve with dependence on their home planets and stars. If humans are limited in how far they can travel in Space away from their Sun due to a lack of exergy-maintaining resources in inter-solar space, then this suggests that other life forms have the same limitations. Thus the theory provides an actual scientific basis of universal law as a potential reason for why they haven’t visited us yet.”
These conclusions are consistent with NASA’s Plan for Sustained Lunar Exploration and Development (released in 2020), the basis for the Artemis Program. In addition to sending astronauts to the Moon for the first time since the Apollo Era, the long-term aim is to establish a program of “sustained lunar exploration” that would result in a surface habitat (Artemis Base Camp) and an orbiting space station (the Lunar Gateway).
The development of BLSS is also vital to NASA’s long-term plans for sending crewed missions to Mars in the next decade — “The Journey to Mars.” These missions are scheduled to commence in 2033 and are expected to culminate in the creation of habitats on the Martian surface. Because of the transit times involved (6 months at least) and the fact that missions can only be launched every 26 months when Mars is in Opposition (closest to Earth), self-sufficiency and ISRU are key aspects of the planning.
While improvements in propulsion technology will allow for shorter transit times and more capable supply chains, self-sufficiency is currently the name of the game for space exploration. As humanity migrates farther away from the cradle, the key to surviving and striving will require that we recognize our dependence on the intricate conditions that spawned us. These include the immediate need for air, water, food, and all the natural systems that create and renew them.
These findings suggest that human migration to space may not be so inevitable. Then again, treating the prospect as something that is either inevitable or not seems a bit naive and inelegant. Instead, we should view it as a potential opportunity that offers extensive benefits and significant challenges in equal measure. To accomplish this migration, we not only need to build the necessary infrastructure and leverage the local resources. We must also ensure that nature’s delicate balance and restorative powers accompany us for the ride!
This article was originally published on Universe Today by Matt Williams. Read the original article here.