Over the last half-century, psychedelics have radically transformed from public enemy number one to the plucky wunderkind promising to transform psychiatry, particularly mental health. Across the globe, ongoing studies are studying how psilocybin (the hallucinogen in “magic mushrooms”), MDMA (better known by its street name, ecstasy), and LSD could help conditions like depression or addiction as well as neurodegenerative conditions like Alzheimer’s.
The great allure of psychedelics is that they provide us with a wide variety of options for fixing brain ailments, especially those like post-traumatic stress disorder, where conventional therapies have fallen short. But herein lies a problem: How exactly psychedelics work is a bit of a mystery. Researchers have theories — psychedelics promote neuroplasticity and disrupt abnormal patterns of brain activity — but the challenging task for scientists is trying to pinpoint the exact brain regions and pathways psychedelics are affecting.
After all, studying the brain is more complicated than it sounds. Mapping the psychedelic effect and observing its microscopic changes requires extrapolating from animal studies and peering into human brains from the safe distance of an MRI scanner. Currently, we can’t observe the brain’s response to a psychedelic in a way that’s up close and personal.
That’s where stem cell biologists like Stevens Rehen come in. At his lab at the D’Or Institute for Research and Education in Brazil, Rehen and his colleagues are turning to a unique tool to open that black box: brain organoids. These tiny quivering masses of brain tissue were first pioneered in 2013 but are quickly becoming a gold standard in neuroscience and psychedelic research. Inverse spoke with Rehen about the research he’s been doing to peel away the mystery.
The interview below has been edited and condensed for clarity.
You’ve been doing stem cell research for quite some time now. What brought you to psychedelics?
My interest in psychedelics began early in my scientific career. My youngest brother is an anthropologist, and he was studying ayahuasca [a psychoactive brew used for spiritual and healing practices], specifically in Santo Daime [a Brazilian religion centered around psychedelic use]. He invited me to attend one of the ceremonies, and the psychedelic experience and altered state of consciousness was very interesting. From then on, I was puzzled about the molecular biology behind the phenomena, which stayed in my mind for over a decade.
Everything clicked in 2013. Madeline Lancaster and Jürgen Knoblich in Austria published the biggest paper on the field of organoids. Some months later, I was invited to attend a Psychedelic Science conference in Oakland, California. Attending that conference made me remember my experience with ayahuasca in the ‘90s and connecting that with the [2013 paper].
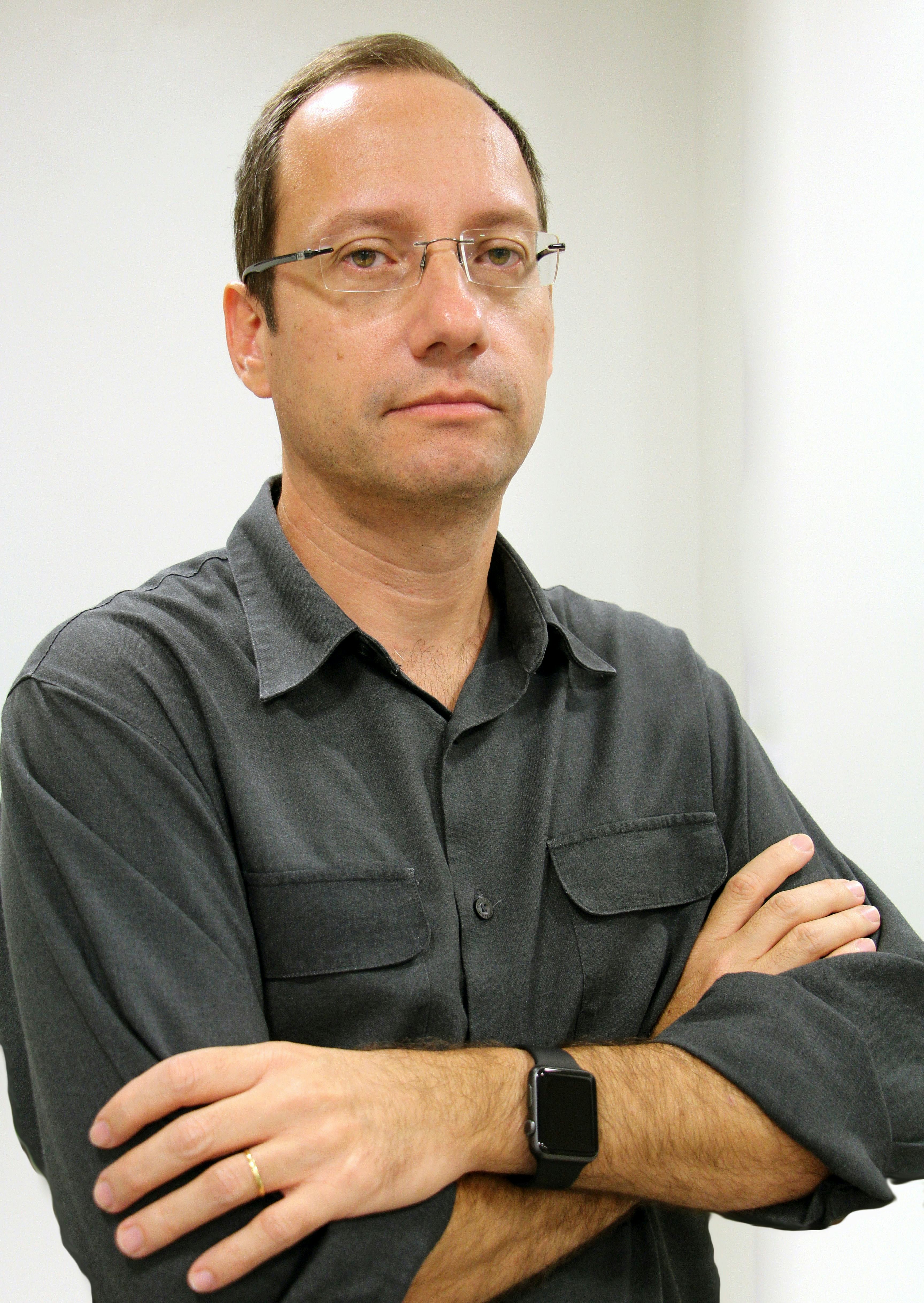
When people imagine brain organoids, they think of mini-brains growing in Petri dishes with the same functionality and structure as our brains. Are brain organoids like our own?
I can understand [how that image] is easier for the broader audience to understand. But it gives the impression, as you said, we’re talking about something with all the functionality of an actual brain, and, of course, that’s not the case.
The magic behind these brain organoids is that these cells interact in a way that’s much closer to what we see in a real brain than in cell cultures. Rather than a simple group of one type of cell in a dish, these structures contain various cells found in the brain and can grow up to five or even 10 millimeters in size. The variety of cell types enables us to observe how these cells interact; for instance, how they help produce neurotransmitters. You can also measure electrical activity, which can be extrapolated to what it would be in an actual brain.
“The magic behind these brain organoids is that these cells interact in a way that’s much closer to what we see in a real brain than in cell cultures.”
What has your research helped illuminate our understanding of psychedelics and their activity in the brain?
The first paper my lab published in 2016 investigated a compound in the ayahuasca brew called harmine. At the time, harmine was being explored as an inhibitor of an enzyme called monoamine oxidase to prevent the degradation of another psychedelic called DMT [also contained in ayahuasca]. Basically, if you have DMT alone, you don’t have any kind of psychedelic experience. But with harmine present, DMT reaches the brain and creates its characteristic psychedelic effect.
We wanted to see if harmine has any other effects on the brain by exposing it to brain organoids. We noticed that it did: it increased neurogenesis, or new neuron growth. This neurogenesis didn’t depend on inhibiting monoamine oxidase but another enzyme called DYRK1A, which is highly expressed in people with Alzheimer’s disease as well as Down syndrome [inhibiting DYRK1A is being explored as a potential therapy for Alzheimer’s and Down syndrome].
Later in 2017, we published a study examining the effect of 5-MeO-DMT — that’s the DMT from certain toads — on brain organoids. Together with my graduate student at the time, Vanja Dakic, we saw that 5-MeO-DMT alters more than a 1,000 proteins found in the brain. We tracked the biochemical pathways associated with these proteins and found a majority of them were involved in neuroplasticity, learning, memory, and even inflammation. Another study we did in 2022 with LSD found more evidence of these drugs influencing neuroplasticity. These findings shed light on the potential therapeutic effects of psychedelics.
Many studies have found this capacity for neuroplasticity may alleviate neurological conditions such as depression and Alzheimer’s disease. But there are still many tweaks to work out. For example, many people with these conditions find the hallucinogenic and other effects of these drugs undesirable. Further, psychedelic trips are unique, which makes studying them hard. How can brain organoids help overcome these challenges?
It comes down to cataloging all the chemicals produced in response to each psychedelic — a molecular signature. Having this kind of signature for each psychedelic, we hope, will lead to a better understanding [of how they work] and finding new therapeutic potentials.
To this end, one of our goals is to create individualized brain organoids. We would give organoids made from someone’s own cells different psychedelics and observe how they respond. However, we have to keep in mind there are limitations. We can’t mimic in the lab the complexities of an individual’s trauma or inflammation from Covid-19 or Alzheimer’s disease in an organoid. We also can’t simulate all the years of people’s lived experiences. But we can try as best we can. We do know the molecules involved in stress, so if we add them to a system, the cells are going to behave, in some sense, similar to what we’ve seen [in a living organism].
Speaking of neuroplasticity, could brain organoid and psychedelic research help us create effective nootropics, aka cognitive enhancers?
Potentially! For example, if we combine psychedelics with these “brains in a dish” platforms where we can follow the changes in electrical activity, we could observe whether there are any improvements or decreases in learning. This, of course, would extend our understanding of neuroplasticity.
So what’s next for your research?
Aside from identifying molecular signatures, I want to use what I learn with brain organoids and apply them to a whole organism. I have a small group in the lab working with Caenorhabditis elegans — the roundworm — to study psychedelics and longevity. We just came out with a preprint where we gave LSD to these animals. They absorbed it and produced metabolites that are very similar to what we see in humans. This sort of research could provide us with a better understanding of the therapeutic potential of LSD.
I’m also interested in developing new tools to follow these neurons within brain organoids, such as using biosensors. Usually, what we have to do to study, for example, the proteins made by these cells, is to kill the cells and fix them with chemicals. But if you create these biosensors, we can see them live in real time and use fluorescent proteins to track any changes. I’m right now collaborating with Promega, a biotechnology company based in Wisconsin, to create this biosensor for organoid research and for my personal goal to study psychedelics better.
Editor’s Note: This story has been updated to reflect the fact that Stevens Rehen’s lab is located at the D’Or Institute for Research and Education in Brazil, not the Federal University of Rio de Janeiro, as previously stated. We are glad to correct the error.