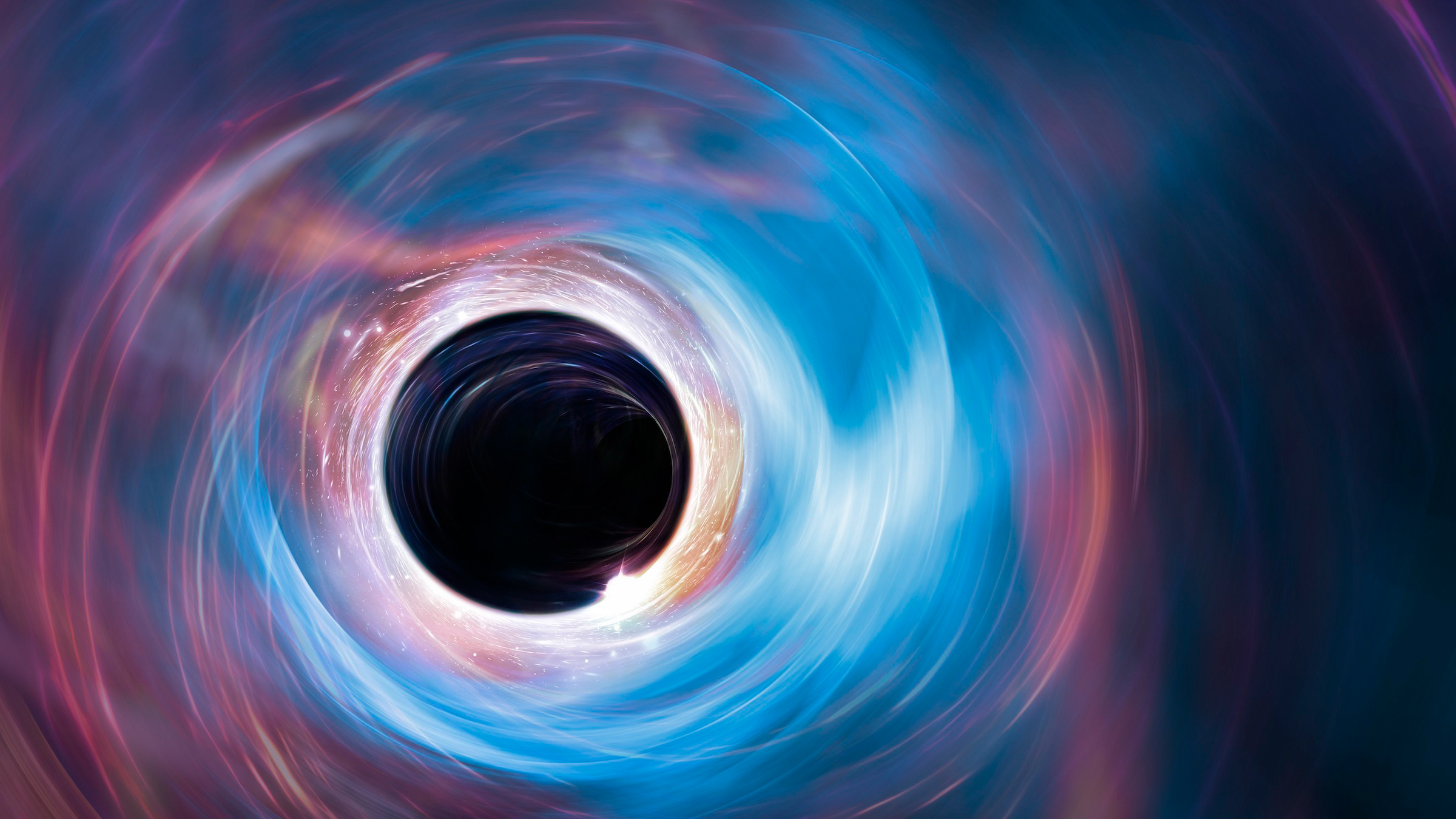
The early universe contained far fewer miniature black holes than previously thought, making the origins of our cosmos's missing matter an even greater mystery, a new study has suggested.
Miniature, or primordial, black holes (PBHs) are black holes thought to have formed in the first fractions of a second after the Big Bang. According to leading theories, these dime-sized singularities popped into existence from rapidly collapsing regions of thick, hot gas.
The pockets of infinitely dense space-time are how many physicists explain the universe's dark matter, a mysterious entity that, despite being completely invisible, makes the universe much heavier than can be explained by the matter we see.
But even though the hypothesis is popular, it has one big problem: we've yet to directly observe any primordial black holes. Now, a new study has offered a possible explanation as to why they didn't form, throwing open cosmology's dark matter problem to wider speculation.
According to the research, the modern universe could have taken shape with far fewer primordial black holes than previous models estimated. The researchers published their findings May 29 in the journal Physical Review Letters.
"Many researchers feel they [primordial black holes] are a strong candidate for dark matter, but there would need to be plenty of them to satisfy that theory," lead author Jason Kristiano, a graduate student in theoretical physics at the University of Tokyo, said in a statement. "They are interesting for other reasons too, as since the recent innovation of gravitational wave astronomy, there have been discoveries of binary black hole mergers, which can be explained if PBHs exist in large numbers. But despite these strong reasons for their expected abundance, we have not seen any directly, and now we have a model which should explain why this is the case."
A hole in the picture
The universe began 13.8 billion years ago with the Big Bang, causing the young cosmos to explode outward due to an invisible force known as dark energy.
As the universe grew, ordinary matter, which interacts with light, congealed around clumps of invisible dark matter to create the first galaxies, connected together by a vast cosmic web. Nowadays, cosmologists think that ordinary matter, dark matter and dark energy make up about 5%, 25% and 70% of the universe’s composition, respectively.
Initially, the universe was opaque, a plasma broth that no light could traverse without being snared by electromagnetic fields produced by moving charges. Yet after 380,000 years of cooling and expansion, the plasma eventually recombined into neutral matter, giving off microwave static that became the universe's first light, the cosmic microwave background (CMB).
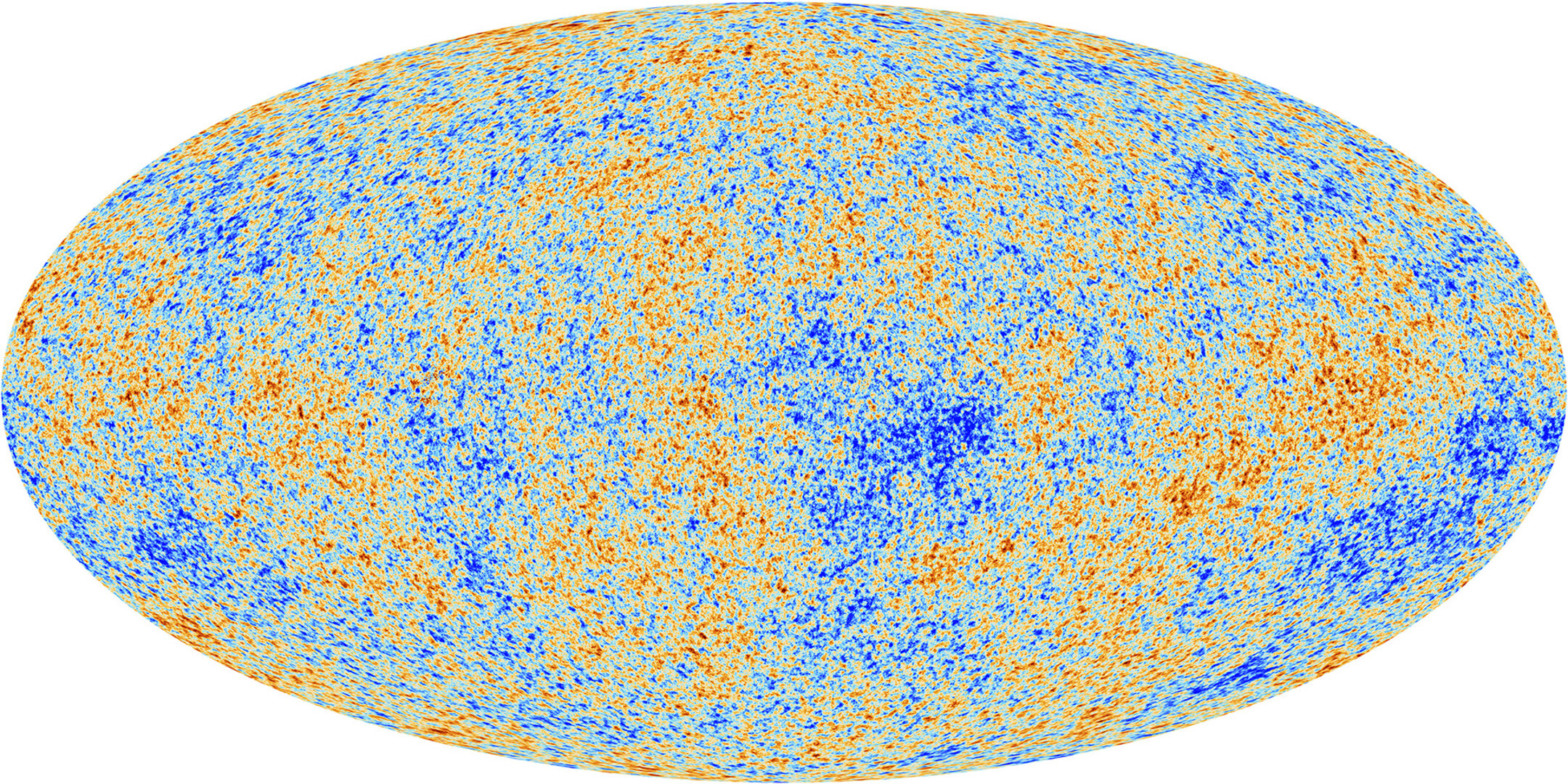
Cosmologists have been searching for these early black holes by studying this first baby picture of the universe. Yet, so far, none have been found.
Some physicists think there's a possibility they haven't discovered the vast numbers of primordial black holes necessary to account for dark matter simply because they've yet to learn how to detect them.
But by applying a model built on an advanced form of quantum mechanics called quantum field theory to the problem, the researchers behind the new study arrived at a different conclusion — we can't find any primordial black holes because most of them simply aren't there.
Primordial black holes are believed to have emerged from the collapse of short but strong gravitational waves rippling across the universe. By applying their model to these waves, the researchers found that it could take much less of these waves to combine than other theories estimate in order to shape larger structures across the universe. And the fewer the waves necessary to recreate the picture, the fewer primordial black holes.
"It is widely believed that the collapse of short but strong wavelengths in the early universe is what creates primordial black holes," said Kristiano. "Our study suggests there should be far fewer PBHs than would be needed if they are indeed a strong candidate for dark matter or gravitational wave events."
To confirm their theory, the researchers will look to future, hyper-sensitive gravitational wave detectors such as the Laser Interferometer Space Antenna (LISA) project, which is due to be sent into space on an Ariane 3 rocket in 2035.