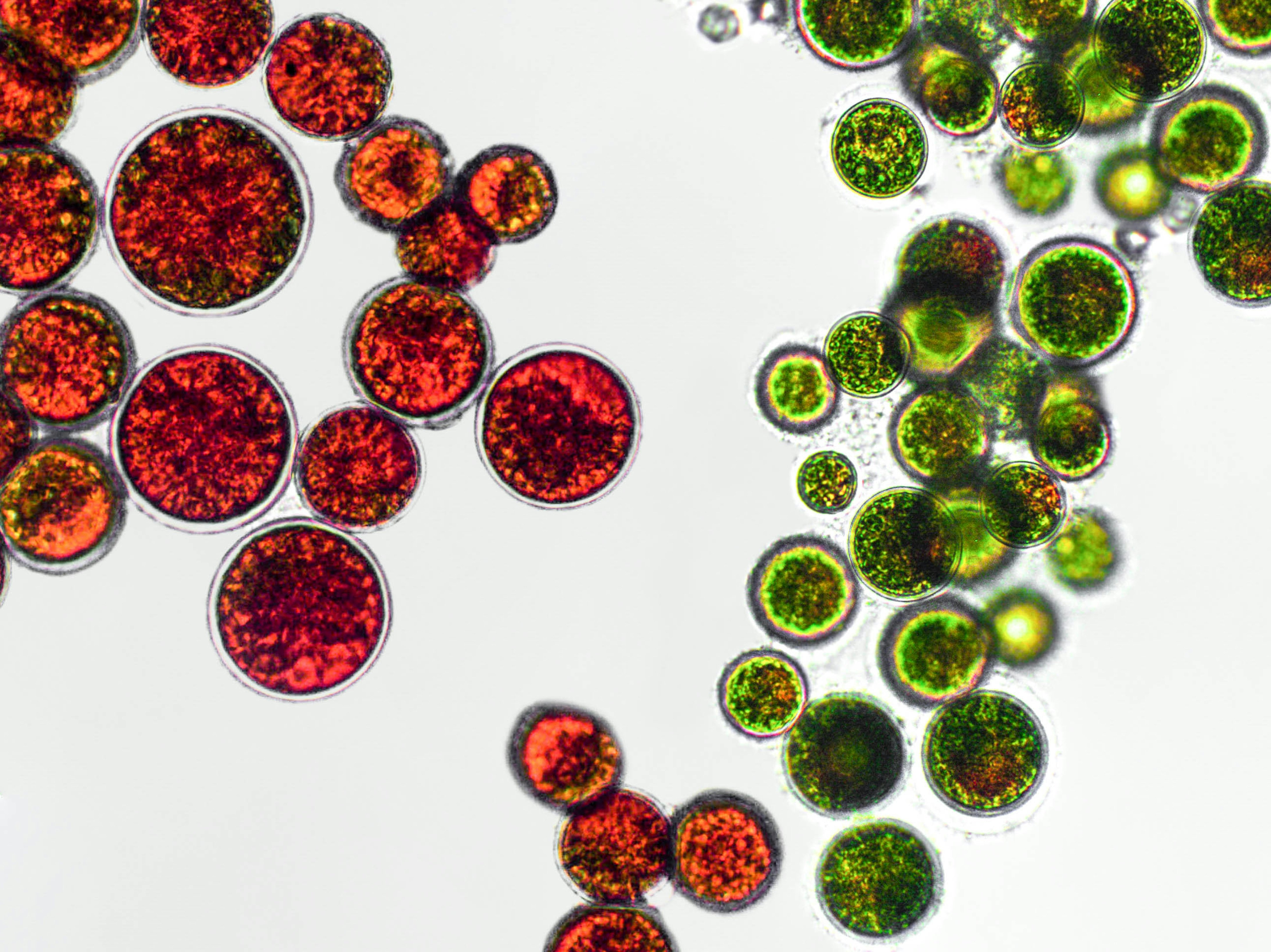
For life on planet Earth, all roads inevitably lead to death, but they’re not all paved the same. This is what Nan Hao, an associate professor in molecular biology at the University of California, San Diego, discovered several years ago. At his lab, Hao spends a lot of time with Saccharomyces cerevisiae — also known as baker’s or brewer’s yeast — studying how these single-celled organisms age, an endeavor that he thinks could inform how humans age, too.
Saccharomyces cerevisiae doesn’t live long, only about one to two weeks. But Hao and his colleagues discovered that during this short span of time, no two yeast cells aged similarly. Some yeast experience a gradual breakdown of their nucleolus, the central, smaller core of the cell’s nucleus that makes a type of RNA called ribosomal RNA (or rRNA). Other Saccharomyces cerevisiae appeared to age when their mitochondria, the cell’s power plant, started to run out of steam. These findings were published in 2020.
Would it be possible, thought Hao, to genetically control these aging pathways to create a third route, one that daresay slows down aging?
In a paper published Thursday in the journal Science, the molecular biologist and his colleagues at UCSD managed to do just that, synthetically constructing what they call a gene oscillator that extended the lifespan of lab grown-yeast cells by 82 percent. It works by creating a negative feedback loop between two genes — SIR2 and HAP4 — controlling nucleolus or mitochondrial aging, reining in the two modes from ever getting ahead of each other in a way that maintains cellular homeostasis more effectively compared to yeast cells without the gene oscillator.
“This is one of the unique synthetic biologies approaches answering the question of how we can regulate aging or how we can control life span,” Alaattin Kaya, a geneticist and assistant professor of biology at Virginia Commonwealth University, who was not involved in the study, tells Inverse.
Engineering an on-and-off longevity switch
In their 2020 paper, Hao and his colleagues found that the SIR2 gene, also known as sirtuin 2 or SIRT2, seemed to be involved in DNA repair whereas the HAP4 gene was a key regulator of mitochondrial function. While the two were separate, they also appeared to naturally mutually inhibit each other, one gene acting as an on-and-off switch for the other.
The goal was to find a way to manipulate this relationship even further, preventing yeast cells from going too much down the nucleolus aging path (which the researchers called mode 1) or the mitochondrial aging path (mode 2). To do this, Hao and his team used computer simulations to design and test out different conformations of genetic circuits involving SIR2 and HAP4.
The design involved a negative feedback loop to stall the aging process by keeping yeast cells sort of stuck, or oscillating, between nucleolus and mitochondria aging (hence the name of the circuit as a gene oscillator).
Once they settled on a promising gene oscillator design, the researchers used synthetic biology to craft and insert it into a group of yeast cells. These mother cells, which, in typical yeast fashion, would bud off to form daughter cells, were then monitored for levels of rDNA silencing (an indicator of nucleolus aging) and heme abundance (an indicator of mitochondrial aging).
In wild-type cells without the gene oscillator, about half of the cells showed a sustained loss of rDNA silencing and ended life in a state with low rDNA silencing and a high abundance of heme. The other cells showed a depletion of heme and ended life in a state with high rDNA silencing and a low abundance of heme.
But in the yeast cells with the gene oscillator, they didn’t commit to either state for a prolonged period during their lifespan, exhibiting short, intermittent pulses between modes 1 and 2. As a result, these synthetically altered yeast cells lived longer than wild-type cells by about 82 percent.
Hao and his colleagues also found that the synthetic gene oscillator was more effective at extending lifespan than just simply overexpressing Sir2 or HAP4 genes. Overexpression of these genes led to variations in gene expression that inevitably drove cells to still commit to either mode of aging and cellular deterioration. The synthetic gene oscillator, on the other hand, maintained cellular homeostasis and extended lifespan more effectively (and healthily).
Can this research be applied to increasing longevity in humans?
Great, so science can help humble yeast cells live longer, but what about humans? Could we put genetic oscillators inside our cells to similarly extend our own lifespans?
Right now, no, since it’s still a proof-of-concept. But with further research, it might be a possibility.
“That’s actually our final goal, the next step,” Hao tells Inverse. “We want to transfer [our research] to different organisms, especially to human cells, and see whether we can apply these same strategies to change their lifespan at the cellular level because that’s relatively straightforward to manipulate and study.”
The researchers are planning to study stem cells and potentially neurons, as well as look at other animal models like mice. This stage will also involve pinpointing all the aging pathways a cell may take aside from modes 1 and 2 found in yeast cells, and the genes involved in those pathways. (Hao says SIR2 is found across many different species but it’s not the only gene controlling longevity, especially in humans.)
It’s important to note, longevity in humans isn’t simply the luck of the genetic draw. Diet, physical activity, the microbiome, as well as socioeconomic factors, and other environmental and lifestyle factors, shape one’s chance of enjoying a longer life. These other largely external factors throw a wrench in engineering a complex biological trait like longevity.
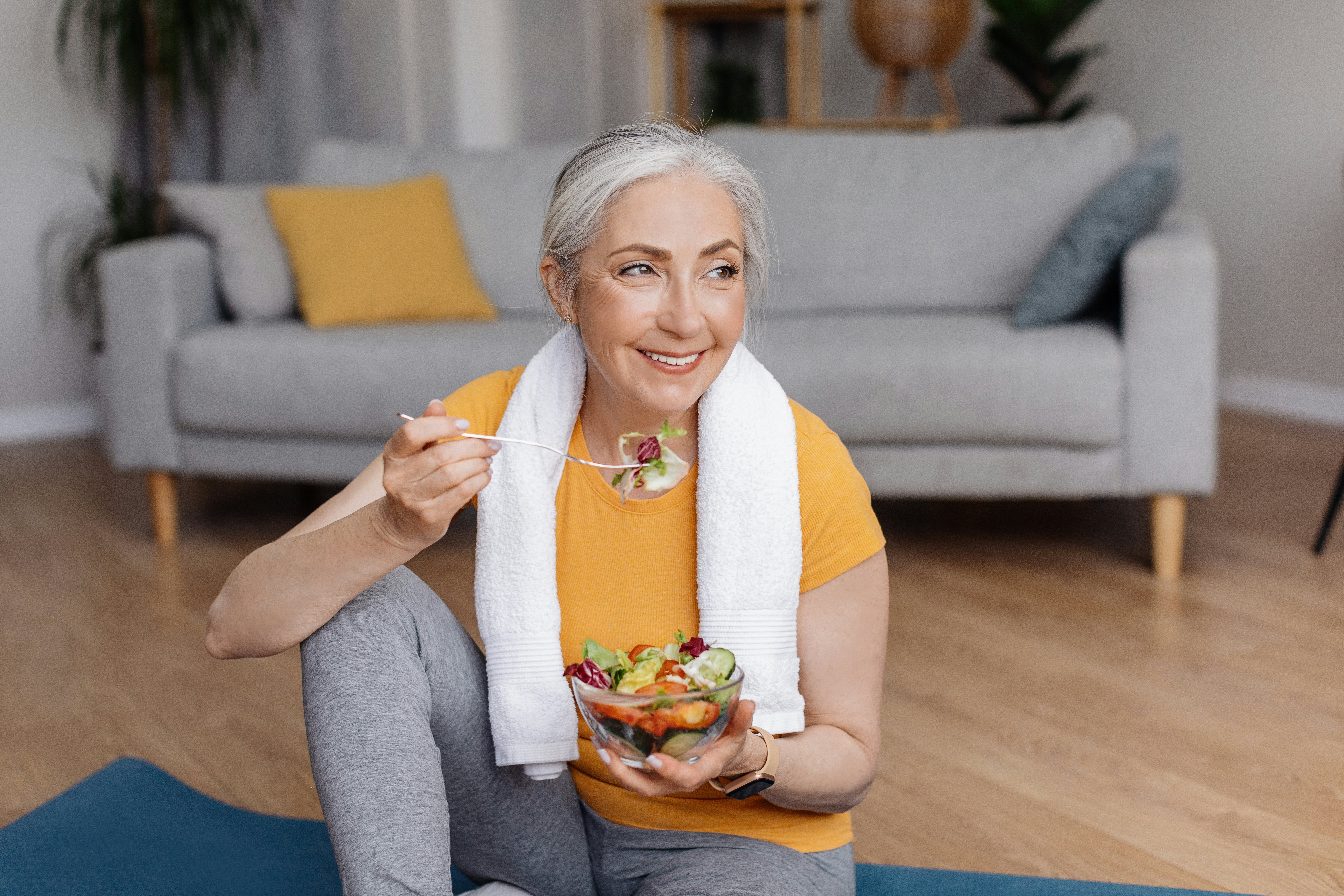
Even if Hao and his team do manage to create long-lived animals or human cells with their genetic oscillator approach, there are still the ethical implications of longevity research that definitely pose an impediment to its application in humans. For example, advances in longevity science risk widening the gaps between the rich and poor in health, wealth, and power, Christopher Wareham, a bioethicist at Utrecht University in the Netherlands, told the Financial Times in January.
Kaya and Hao say even if we can’t use the genetic oscillators for longevity, we could use them to help prevent or treat age-related diseases like neurodegeneration or mitochondrial dysfunction where, with age, DNA unique to the mitochondria (called mitochondrial DNA) accumulates mutations and the organelle, on the whole, produces more reactive oxygen species that cause more cellular damage.
“Genetic oscillators can be very robust to maintaining homeostasis although they keep changing but they’re changing within a narrow range,” says Hao.
There’s also still the question of why exactly this oscillation between the two different aging modes works — namely, the exact biochemical mechanism keeping aging at bay. Kaya believes it could the mini-breaks between oscillations exposes the cells to low doses of stress, which may encourage cell growth and longevity.
Hao acknowledges low levels of stress can do that to cells but he doesn’t believe this is the case here since the pace at which the engineered yeast cells multiplied was a bit faster than the non-engineered wild-type yeast cells. That, along with how the oscillator affects other yeast cell genes and cellular functions potentially outside of aging, is an avenue of future research.