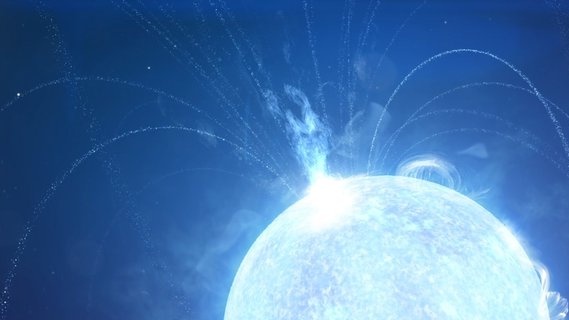
Scientists may finally understand the dynamics of neutron star "glitches" that occur when these ultradense dead stars suddenly speed up their spins. It would appear that the strange behavior may be caused when tiny vortices of swirling inner material "break the surface" of these intense stellar corpses.
The new breakthrough in understanding neutron star behavior interestingly comes from a unified team of astrophysicists and quantum physicists — who typically study interactions that govern the subatomic world — studying an exotic form of matter here on Earth.
A better understanding of the neutron star glitches could reveal more about their inner composition and movements, thus giving scientists a window into an object that's made of arguably the universe's most unique and strange form of matter. Neutron stars, in essence, are made almost exclusively of neutrons, which is why they're so utterly dense.
"Our research establishes a strong link between quantum mechanics and astrophysics and provides a new perspective on the inner nature of neutron stars," research lead author and Innsbruck University researcher Elena Poli said in a statement.
Related: Quantum chemistry experiment on ISS creates exotic 5th state of matter
Bringing neutron stars down to Earth
Neutron stars are formed when massive stars "die" and their stellar cores, with masses between one and two times that of the sun, collapse to the width of just 12 miles (20 kilometers). That's an absolutely huge reduction in size. The neutron-rich matter that comprises neutron stars is so dense that a mere sugar cube of it would weigh about 1 billion tons on Earth — about 150 times the weight of the Great Pyramid of Giza.
That extreme weight, along with the immense distance to these stellar corpses, means we can hardly bring neutron star samples down to Earth to study. However, the multidisciplinary team was able to indeed bring the study of neutron stars "down to Earth" by numerically simulating a neutron star using a proxy in the form of ultracold dipolar atoms — an exotic phase of magnetic gases with a negatively charged atom coupled to a positively charged atom over long distances.
The glitching of neutron stars may suggest that the matter beneath these objects' surfaces exists in the form of a superfluid, a substance that resembles a liquid but has zero viscosity — a measure of the resistance a fluid has to changes in shape or to flowing around.
High-viscosity fluids, such as honey or cold maple syrup, flow slowly and can even act like solids. Think, stiff peanut butter or even glass. Low-viscosity fluids, on the other hand, flow more rapidly. But zero-viscosity superfluids are another story. They rotate in the form of numerous tiny swirling vortices, all of which carry a little bit of the system's angular momentum.
A key factor in this behavior and thus a vital element of neutron star glitching would be a state that shows both crystalline and superfluid properties — a so-called "supersolid." If neutron stars demonstrate this behavior as they rotate, often as fast as hundreds of times per second, glitches would occur as vortices break out of the star’s inner crust — the superfluid — to its solid crystalline outer crust. Those flyaway vortices would carry with them angular momentum that boosts the rotational speed of the star's outer layer.
This supersolid phase has been induced in ultracold dipolar atoms of Erbium (Er) and Dysprosium (Dy) by a group led by Francesca Ferlaino at the University of Innsbruck, also an author of this work.
The team found that glitches can indeed occur in ultracold supersolids, which are analogous to larger, more extreme glitching demonstrated by neutron stars. These results suggest that it is truly superfluid vortices carrying angular momentum to the surface of these stars that make them appear to glitch.
The approach developed by the multidisciplinary team will now be explored further by investigating the glitch mechanism in more detail as well as how it might depend on the quality of the supersolid material. The study could also help pioneer a new way to investigate stellar remnants like neutron stars in the lab for other purposes.
"This research shows a new approach to gain insights into the behavior of neutron stars and opens new avenues for the quantum simulation of stellar objects from low-energy Earth laboratories," Ferlaino concluded.
The team's research was published in November in the journal Physical Review Letters.