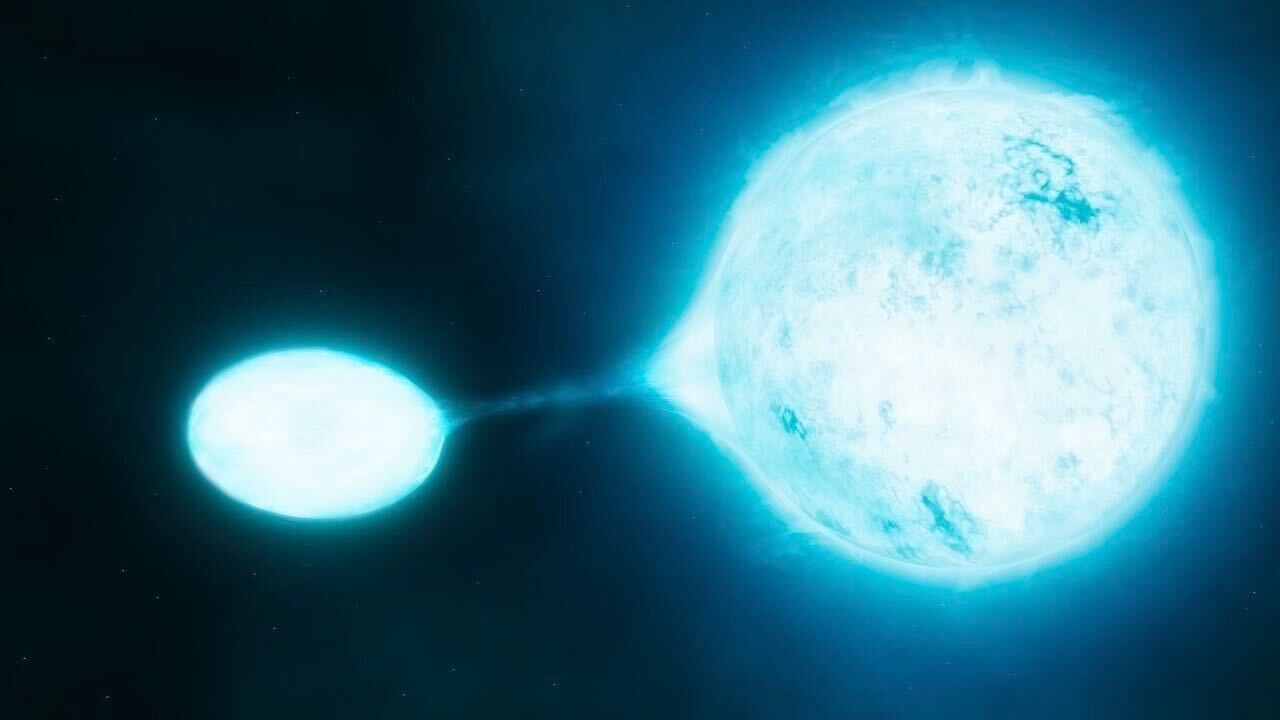
Black holes have a preference for forming around two "universal" masses equivalent to about nine and 16 times the mass of our sun, according to a new study of the frequency of the gravitational-wave ‘chirps’ released when two black holes collide and merge. These findings could ultimately pave the way for an independent measure of the expansion of the Universe.
Since 2015, 90 gravitational-wave events have been identified by detectors at sites specifically built to find these information-rich ripples in spacetime. This includes laboratories such as the Laser Interferometer Gravitational-wave Observatory (LIGO) in the US, its sister site, Virgo, in Italy and the Kamioka Gravitational-Wave Detector (KAGRA) in Japan. Each merger produces what's known as a chirp, which is a blast of gravitational waves that rapidly increase in frequency as two black holes spiral closer and closer around one another before colliding and merging. The frequency and amplitude of this chirp is connected to the mass of the black holes that have merged; their combined mass is sometimes referred to as the "chirp mass."
"When two black holes merge, they produce gravitational waves that can be 'heard' on Earth," Eva Laplace, an astrophysicist at the Heidelberg Institute for Theoretical Studies in Germany and an author on the study, told Space.com. "By listening to these chirps and analyzing them, it is possible to measure the combined mass of distant merging black holes."
Stellar-mass black holes form during the death of a massive star. While in some cases a massive star will explode as a supernova and leave behind a compact neutron star, in other cases there is no explosion. Instead, the star's core collapses under gravity so severely it forms a black hole that eventually causes the rest of the star to cave in around it.
The masses of these resulting black holes determine the frequency of the gravitational-wave chirp that is emitted when they merge, and are also related to the mass of the stars that formed them. One would therefore expect a wide range of stellar mass-black holes to exist in the universe, reflecting the various masses of their progenitor stars, and indeed this is mostly the case. However, astronomers have been baffled to find more black holes associated with gravitational-wave events that have masses around 8–9 solar masses and 14–16 solar masses, but for some reason, hardly any with masses in between.
Now, new research conducted by Laplace alongside fellow astrophysicists Fabian Schneider, and Philip Podsiadlowski, also of the Heidelberg Institute for Theoretical Studies in Germany, addresses this apparent preference for merging black holes to converge on certain masses over others.
"What our study shows is that there is always a gap in black-hole masses between 9 and 16 solar masses,” Schneider told Space.com.
What happens inside massive stars
The existence of the mass gap is dictated by what is happening inside a massive star as it nears the end of its life.
Young stars "burn" hydrogen in their cores via their intrinsic nuclear fusion processes; in massive stars, the dominant version of this process is known as the carbon–nitrogen–oxygen (CNO) cycle. This refers to a long chain of reactions involving hydrogen, plus those elements, that eventually produce helium and release a lot of energy to power the star. However, once the star’s core runs out of hydrogen, its energy production falters. Without enough energy to hold the star up, the core begins to contract under gravity. This increases the core's temperature by millions of degrees Celsius, until it's hot and dense enough to begin burning helium and temporarily halt the contraction.
At this stage, the star is like an onion, with various layers. Its core is burning helium. Around the core is a non-burning layer of helium, and ordinarily, around that is a shell that’s still burning some leftover hydrogen to produce even more helium that sinks to the stellar core. This extra helium further increases the core’s mass and temperature, speeding up the nuclear reactions which control the star’s evolution. Ultimately, this leads to a supernova and usually either a neutron star or a lone black hole, depending upon the compactness of the star’s core (in the case of stars with 130-250 solar masses and fairly primitive chemical compositions, they can sometimes explode and utterly destroy themselves in a so-called pair-instability supernova, leaving nothing behind).
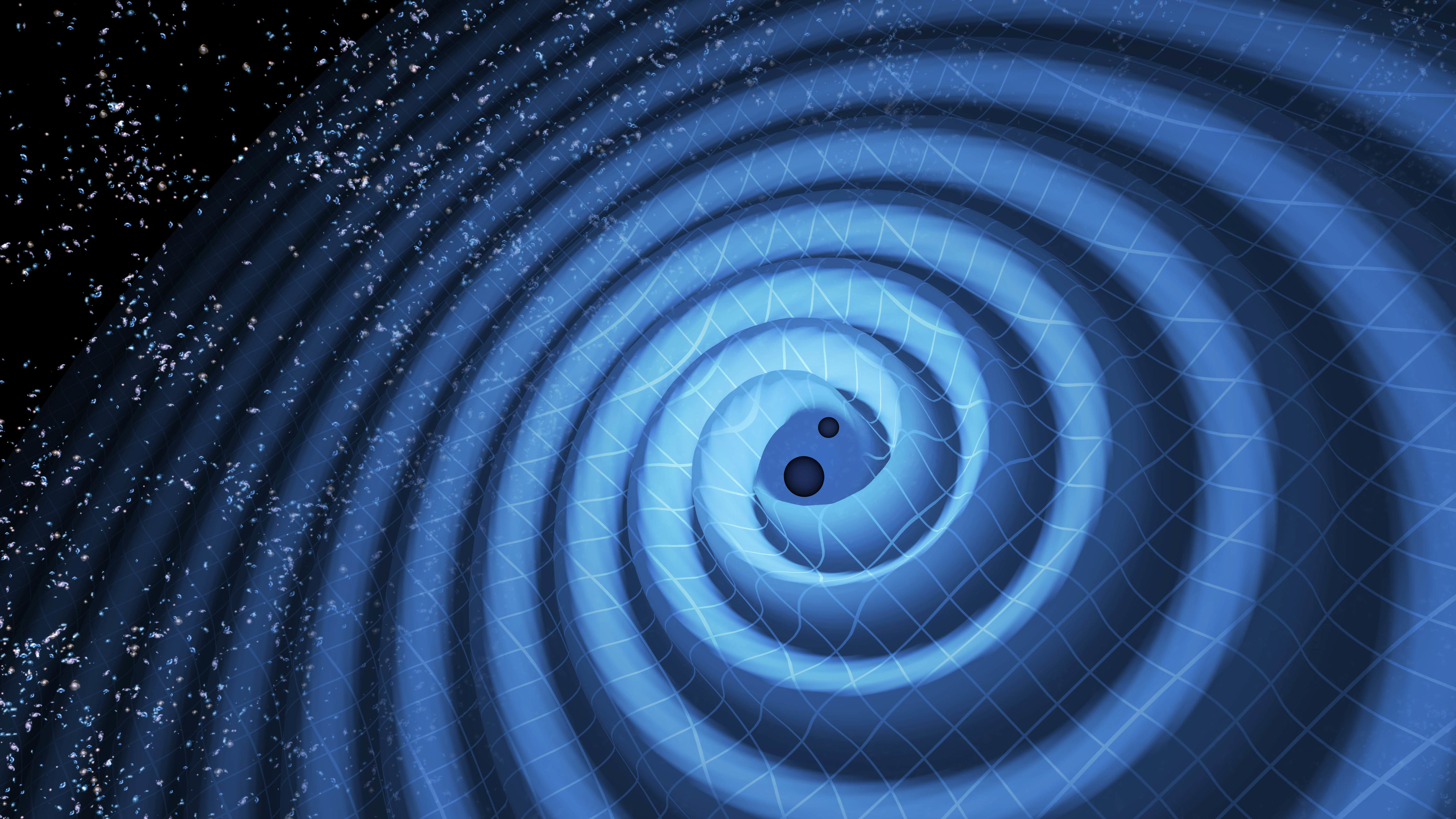
By contrast, black-hole mergers are the product of massive binary star systems. During the time that they still exist as stars, the close companions are able to steal matter from each other, stripping away each other’s hydrogen-burning shell. Without this shell, a star’s core doesn’t gain that extra helium, which changes the star’s evolutionary trajectory. Conditions inside the core of a star that has lost its hydrogen shell are such that thermal neutrinos – tiny, ghost-like particles that spontaneously form – escape the star, carrying some of the core’s thermal energy with them. This lowers the temperature of the core and slows down nuclear reactions. The result is a decrease in energy production which allows the core to gravitationally contract some more. This leads to a very dense core that, when the star exhausts all its nuclear fuel and dies, can collapse to form a black hole.
In a binary system, this can lead to two black holes that eventually merge with a chirp of gravitational waves.
“Because of a complex interplay between neutrino losses, nuclear burning and core contraction, we find that stars of specific core masses are more prone to collapsing to black holes rather than exploding as a supernova and leaving behind a neutron star,” said Schneider.
This interplay leads to common black hole masses, according to the calculations of Schneider, Laplace and Podsiadlowski. In their models, the black hole masses tend to converge on two values, which are 9 and 16 times the mass of our Sun. These values are very close to the peaks that have been observed in the gravitational-wave data, which are at about 8 and 14 solar masses, so they don't exactly match, but remain within observational uncertainties.
Measuring the expansion of the universe
A prevalence of certain masses of black hole not only tells us about the physics of massive stars, but it also gives astronomers another way to measure the expansion rate of the universe, known as the Hubble constant. This has come under the spotlight in recent years because different methods give contradictory values for the Hubble constant.
The frequency of a gravitational-wave chirp depends mostly on the combined masses of the black holes involved, but a portion of it is also connected to their redshift, which tells us their distance, because the farther away they are, the more the expansion of the universe has shifted them to longer wavelengths.
Until now, it had been impossible to disentangle the black hole masses from the redshift in the chirp. However, knowing that a large proportion of black holes have these universal masses gives scientists an advantage.
“We can then take a statistical approach to decouple the masses from the redshift,” said Schneider. This technique would require a larger sample of gravitational-wave events than we currently have, but in principle, would provide a means of measuring the Hubble constant from the redshift that is independent of methods involving standard candles such as Type Ia supernovae.
A larger sample of gravitational-wave events may be coming soon. A new observing run involving LIGO, Virgo and KAGRA that will last 20 months has recently begun, and the aim is to discover another 300 events. We will know soon enough whether the new results enhance the peaks in the distribution around the universal masses and the gap between them.
The findings were published in The Astrophysical Journal Letters.