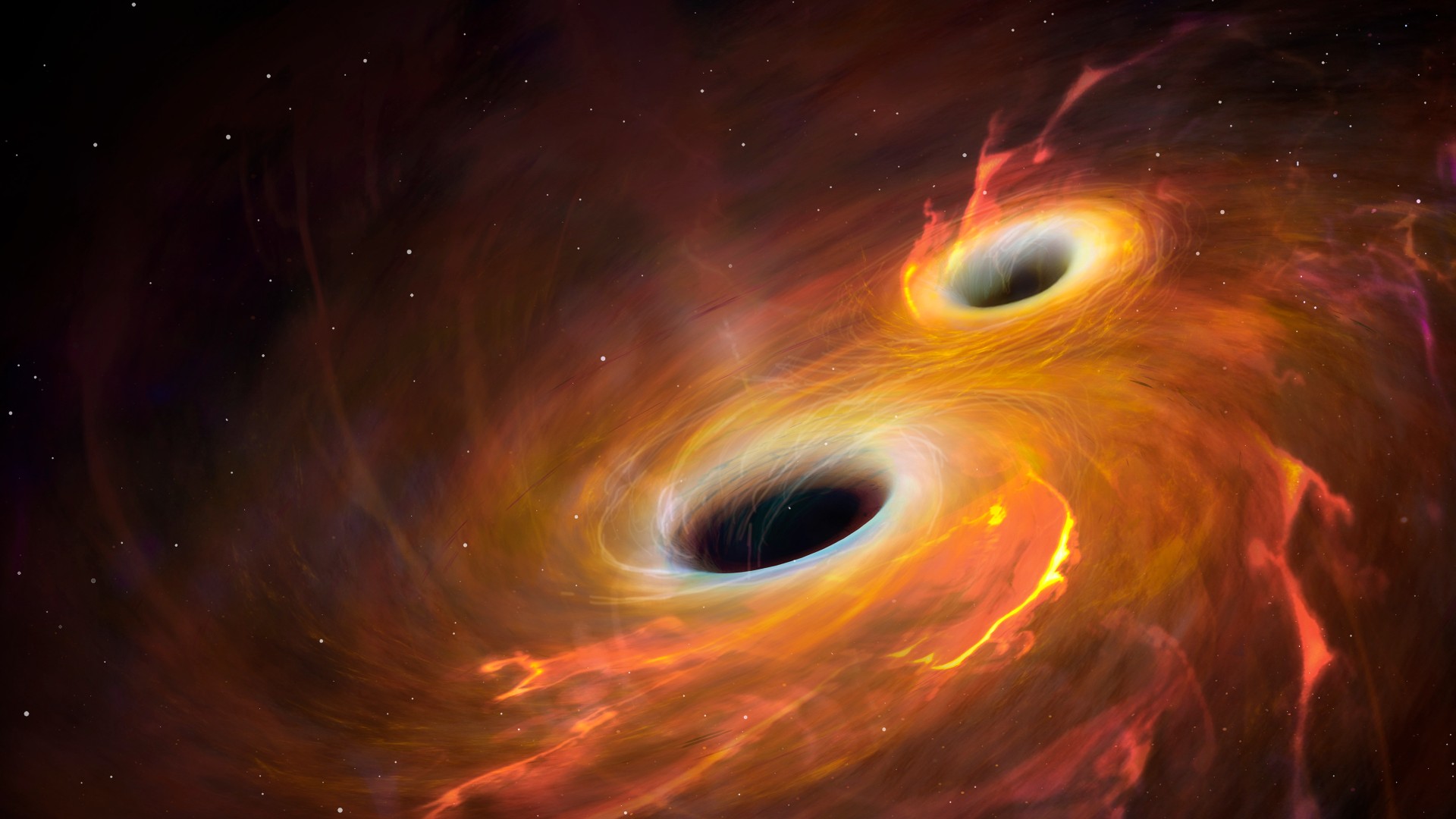
On May 24, scientists at the Laser Interferometer Gravitational-Wave Observatory (LIGO) began an 18-month campaign to detect the most distant collisions between black holes and neutron stars ever observed.
"We're very excited," said Michael Landry, head of LIGO Hanford Observatory.
Thanks to upgrades, LIGO's detectors are now so sensitive they can measure gravitational waves — ripples in the fabric of space-time first predicted by Albert Einstein — emitted from colliding black holes in galaxies more than 5 billion light-years from Earth.
The hunt for gravitational waves will be extended into a worldwide effort. Later in the campaign, LIGO's two U.S. facilities in Hanford, Washington, and Livingston, Louisiana, will be joined by the Virgo detector in Italy and the Kamioka Gravitational Wave Detector (KAGRA) in Japan.
Studying the black hole population
Black holes are bottomless pits in space-time where gravity has become so strong that not even light can escape. Just as planets can orbit stars, and stars can move around other stars, so black holes can circle one another.
"A black hole warps space-time, and when they circle around each other they create ripples in space-time," said Sheila Dwyer, staff scientist at LIGO Hanford.
These ripples, or gravitational waves, carry energy and angular momentum away from the two black holes, causing them to spiral closer and closer toward each other until they eventually collide in one of the most energetic events in the universe.
In September 2015, LIGO detected the first gravitational waves ever observed.
"I will remember that day for the rest of my life. It was a great moment," Landry said.
The waves were emitted by two black holes with masses roughly 30 times that of the sun. These cosmic monsters crashed into each other at nearly half the speed of light, at a distance more than 1 billion light-years from Earth.
LIGO detects the gravitational waves emitted by such collisions by observing the minute displacement of mirrors that hang at the end of two long tunnels. As a gravitational wave passes through LIGO's facilities, the positions of the mirrors shift by less than the size of a proton. Such miniscule displacements can be measured with the help of laser beams that bounce off the mirrors.
"The mirrors have to be a factor of 10 billion times more still than the ground at Hanford that we are standing on," Landry told Live Science. "This is a really difficult experiment, and reaching this goal is one of the most difficult parts of it."
After several decades of struggles to overcome technological challenges, the chief architects behind LIGO — Kip Thorne, Rainer Weiss and Barry C. Barish — were awarded the Nobel Prize in Physics in 2017 for the first gravitational wave detection.
Since this initial detection, LIGO and Virgo have observed 90 gravitational wave events created by colliding black holes or neutron stars, which are highly compact remnants of massive stars that have exploded in a supernova.
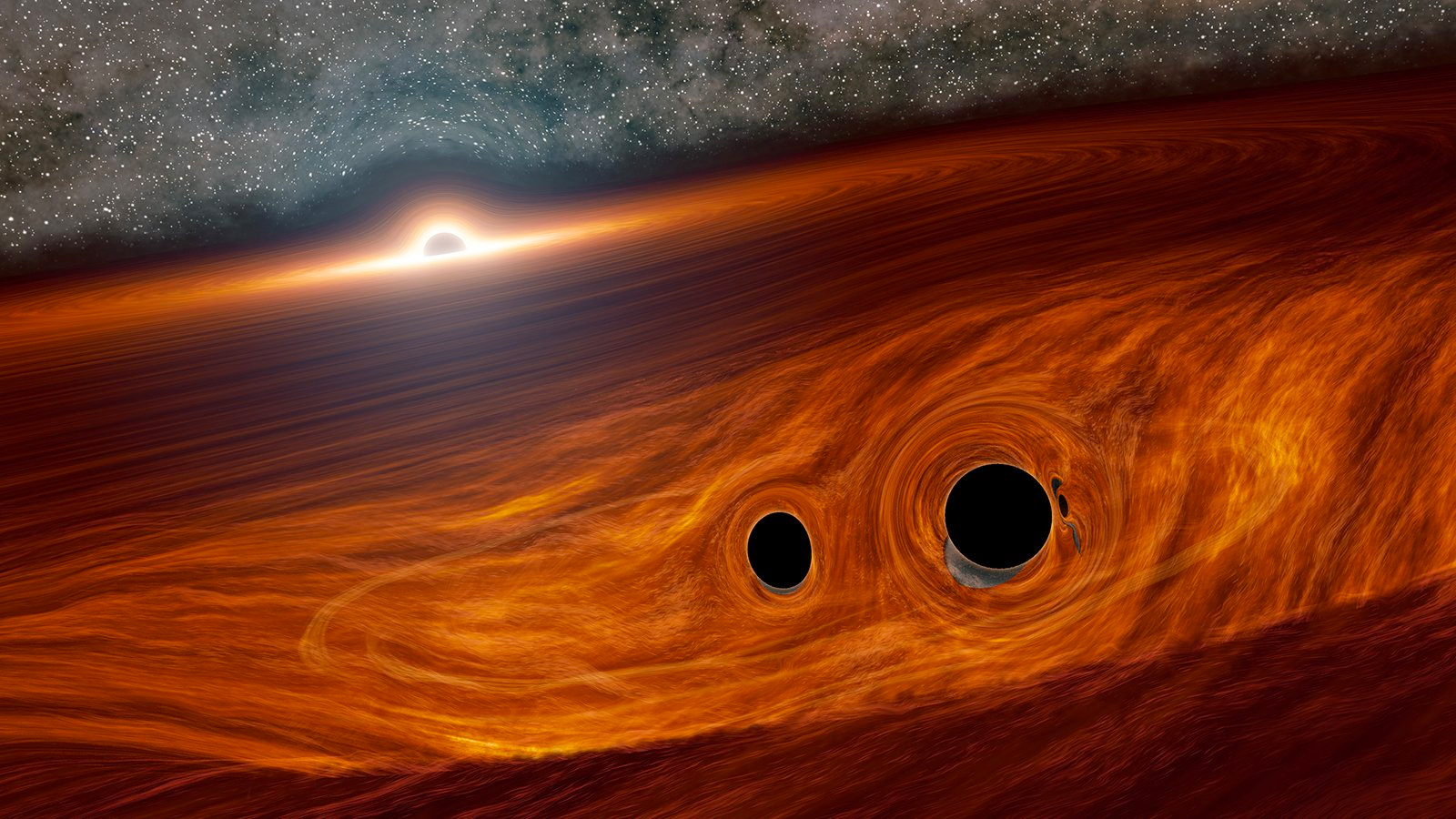
Even more detections are forthcoming thanks to the upgraded instruments. ”We’re going to get several hundreds of events in this 18-month-long run,” Landry said.
Thanks to these detections, astronomers can study not only single black hole pairs but the entire population of such pairs.
"We will be able to pin down the origin of these binary black holes," explained Landry. "Do the black hole binaries come from two stars that were born together and then lived through two successive supernovae? Or are they objects that were captured around one another?"
In 2017, LIGO detected the extraordinary collision of two neutron stars. While LIGO and Virgo detected the gravitational waves from the collision, telescopes around Earth observed the electromagnetic radiation. This was the first time that both gravitational waves and electromagnetic radiation were observed simultaneously from the same source. Using these observations, researchers could study how colliding neutron stars form a kind of cosmic nuclear laboratory where elements heavier than iron are created.
For the upcoming observations, new kinds of sources might be detected. "This observation run is our best chance to date to see a new source of gravitational waves, such as an isolated neutron star in our galaxy," Landry said. "It would produce a continuous gravitational wave signal that’s present for the lifetime of the experiment. If we detect that, it would be a huge deal."
Upgraded LIGO
On May 18, LIGO gave scientists a preview of what is possible with the new upgrades, when it detected what may be a collision between a neutron star and a black hole. This detection occurred even though LIGO's facilities were in what's known as an "engineering run," which means that tweaks to the instrumental setup can still be made by LIGO’s engineers before the start of the observations.
In the coming months, LIGO is projected to measure at least one event from colliding black holes and neutron stars every week. Future upgrades towards the end of this decade will make it possible for LIGO to observe a few such events every day. Recently, the Indian Cabinet approved the construction of a gravitational wave detector in India.
"With the addition of LIGO India in the early 2030s, we will have five observatories worldwide, which will be fantastic," Landry said.
New types of observatories are also planned — the Cosmic Explorer in the U.S. and the Einstein Telescope in Europe — that promise the detection of even more distant black hole mergers.
"If the detectors are a factor of 10 more sensitive, we could look back to the very first stars and see all the stellar mass black hole mergers in the history of the universe," said Sheila Dwyer. "It’s kind of mindblowing."